Contributed by Kisung Ko
Ko, K. 2000. Using antimicrobial proteins to enhance plant resistance. APSnet Features. Online. doi: 10.1094/APSnetFeature-2000-0500C
Plants have both structural and biochemical defense strategies against pathogens. Plant pathogens, in turn, have counter strategies to ensure successful infection. Plant disease results when interactions between plants and pathogens leads to abnormal growth or crop yield. Plants grown for food, fiber, forage, and ornamental purposes may be severely damaged and killed by diseases caused by pathogens. Chemical and biological treatments, cultural practices, and resistant cultivars are used to control plant diseases and prevent severe crop losses. Unfortunately, these activities are not always successful.
For instance, in one of the most widely cultivated fruit crops, apple, certain varieties are preferred by consumers and farmers for their fruit qualities and orchard characteristics. However, most of these ‘accepted’ apple varieties are susceptible to diseases, and disease control is dependent on pesticides. Conventional plant breeding for single trait disease resistance in a perennial crop such as apple is hindered by self-incompatibility and heterozygosity. In other words, apples, as well as many other plants, do not breed true to variety. Even though they are disease resistant, progeny trees and plants often lack the table quality of the parents and are not accepted by consumers. Recent advances in genetic engineering offer alternative ways to transfer a resistance gene into popular commercial varieties without changing other favorable traits.
One approach to improve a plants' defense against a particular pathogen that has been made possible by genetic engineering is to use genes found in fungi, insects, and animals. Antimicrobial proteins, peptides, and lysozymes that naturally occur in insects (Jaynes et al. 1987), plants (Broekaert et al. 1997), animals (Vunnam et al. 1997), and humans (Mitra and Zhang 1994, Nakajima et al. 1997) are now a potential source of plant resistance.
The production of active oxygen species like superoxide anions, hydroxy radicals and hydrogen peroxide, H2O2, have been observed in many plant-pathogen interactions and are known to play an important role in plant defense. Plants have been engineered to continuously produce active oxygen species. In transgenic potatoes containing a H2O2-generating glucose oxidase gene from the fungus Aspergillus niger, the resulting apoplastic accumulation of peroxide ions enhanced the plants resistance to Phytophtora infestans, late blight; Verticillium dahliae, Verticillium wilt; and Alternaria solani, early blight (Wu et al. 1997).
 Figure 3.1 An apple tree severely infected with fire blight caused by the bacterium Erwinia amylovora. Note the brown dead shoots killed by the bacteria among the green foliage. Photo courtesy of Kisung Ko, Cornell University.
|
Lytic peptides are small proteins with an amphipathic a-helical structure which makes pores in membranes resulting in the lysis, for example, of the bacterial cell membrane (Boman 1991). Cecropins are antibacterial lytic peptides native to the hemolymph of Hyalophora cecropia, the giant silk moth. Transgenic tobacco plants expressing cecropins have increased resistance to Pseudomonas syringae pv. tabaci, the cause of tobacco wildfire, a devastating disease that is difficult to control (Huang et al. 1997). Bacterial blackleg of potato caused by Erwinia carotovora subsp. atroseptica can result in 30% yield reduction and 25% loss in storage even though chemical treatments and breeding for resistance are practiced. Synthetic lytic peptide analogs, Shiva-1 and SB-37, produced from transgenes in potato plants reduce bacterial infection caused by E. carotovora subsp. atroseptica in transgenic potato plants (Arce et al. 1999). Fire blight, a bacterial disease of apple caused by E. amylovora (Figure 3.1) is hard to control because of limited effectiveness of antibiotic sprays, the development of antibiotic-resistant bacterial populations in orchards, and lack of commercially acceptable fire blight resistant varieties. Transgenic apple expressing the SB-37 lytic peptide analog showed increased resistance to E. amylovora in field tests (Norelli et al. 1998).
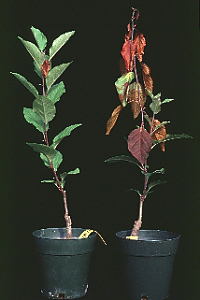 Figure 3.2 Transgenic ‘Royal Gala’ apple line (left) expressing attacin has significantly enhanced resistance to fire blight compared to the non-transgenic (right). Photo courtesy of Herb S. Aldwinckle, Cornell University.
|
Attacins are another group of antibacterial proteins produced by H. cecropia pupae (Hultmark et al. 1983). The mechanisms of antibacterial activity of this protein are to inhibit the synthesis of the outer membrane protein in gram negative bacteria (Carlsson et al. 1998). Attacin expressed in transgenic potato enhanced its resistance to bacterial infection by E. carotovora subsp. atrospetica (Arce et al. 1999). Transgenic pear and apple expressing attacin genes have significantly enhanced resistance to E. amylovora in in vitro and growth chamber tests (Figure 3.2) (Norelli et al. 1994, Reynoird et al. 1999, Ko et al. 2000). In field tests, reduction of fire blight disease has been observed in transgenic apples expressing attacin genes (Norelli et al. 1999). Transgenic apple expressing attacin targeted to the intercellular space, where E. amylovora multiplies before infection, has significantly reduced fire blight, even in apple plants with low attacin production levels (Ko et al. 2000).
Lysozymes are enzymes that hydrolyze the peptidoglycan layer of the bacterial cell wall. Hen egg-white lysozyme (HEWL), T4 lysozyme (T4L), and human lysozyme genes have been cloned and transferred to enhance plant bacterial or fungal resistance. These lysozyme genes have been used to confer disease resistance against plant pathogenic bacteria in transgenic tobacco plants (Trudel et al. 1995, Kato et al. 1998). T4L, from T4-bacteriophage, also has been reported to enhance resistance in transgenic potato against E. carotovora, which causes bacterial soft rot (Düring et al. 1993). Transgenic apple plants with the T4L gene showed significant resistance to fire blight infection (Ko 1999). Human lysozyme transgenes have conferred disease resistance in tobacco through inhibition of fungal and bacterial growth, suggesting the possible use of the human lysozyme gene for controlling plant disease (Nakajima et al. 1997).
The fungus Trichoderma harzianum is a biological control agent that has antagonistic activity against phytopathogenic fungi. The mechanism of this activity is to inhibit spore germination and germ-tube elongation, and to degrade the tips of fungal hyphae. To achieve this, Trichoderma produces enzymes that catalyze the hydrolysis of chitin in the fungal cell wall. Chitinases can be classified as endochitinase, exochitinase (N-acetyl-b -D-glucosaminidase), and chitobiosidase (Tronsmo and Harman 1993). Transgenic potato plants expressing the endochitinase gene showed resistance to the pathogens A. solani (early blight) and B. cinerea (gray mold) (Lorito et al. 1998). Venturia inaequalis, the fungus causing apple scab, infects apple leaves and fruits causing reductions in fruit productivity, marketability, and shelf life (Figure 3.3). Multiple applications of fungicides are relied upon by apple growers almost exclusively to control this disease during the growing season. Transgenic 'McIntosh' apple trees expressing either the endo- or exochitinase gene or both genes have increased resistance to apple scab (Figure 3.4) (Bolar et al. 1999, Bolar 1999). These results suggest the potential broad usage of chitinase transgenes to control fungal diseases of plants.

|
Figure 3.3 Scab infected apple leaves (A) and fruit (B). Trees can be defoliated by the leaf infections and scabby fruits are rejected by consumers and have poor processing and storage qualities. Photos courtesy of David M. Gadoury, Cornell University. |
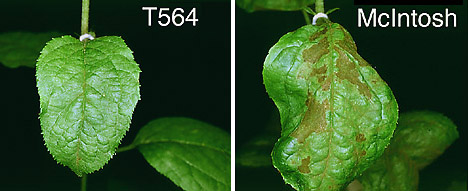 |
Figure 3.4 Scab lesions on a leaf of a transgenic ‘McIntosh’ line T564 (A) compared to those on susceptible non-transgenic ‘McIntosh’ leaf (B). Photos courtesy of Jyothi Prakash Bolar, Cornell University. |
Since most cultivated plants are either consumed fresh or processed for human or animal consumption, it is a given that transgenic crops expressing non-plant genes must utilize genes whose proteins are non-allergenic and non-toxic, both to those consuming them and to non-target organisms. New conventionally bred cultivars of certain crops (ex. potato, tomato, and cucurbits) that have the potential to produce toxic concentrations of natural products should also be checked for toxicity. Transgenic varieties of these crops should be handled similarly.
References
Arce, P., Moreno, M., Gutierrez, M.,Gebauer, M., Dell'Orto, P., Torres, H., Acuna, I., Oliger, P., Venegas, A., Jordana, X., Kalazich, J., Holuigue, L. 1999. Enhanced resistance to bacterial infection by Erwinia carotovora subsp. atroseptica in transgenic potato plants expressing the attacin or the cecropin SB-37 genes. American Journal of Potato Research 76: 169-177.
Bolar, J.B., Norelli, J.L., Wong, K.W., Hayes, C.K., Harman, G.E., and Aldwinckle, H.S. 1999. Expression of endochitinase from Trichoderma harzianum in transgenic apple increases resistance to apple scab and stunts growth. Phytopathology 90:72-77.
Bolar, J.B. 1999. Genetic engineering of 'McIntosh' apple for resistance to scab (Venturia inaequalis). Ph.D Dissertation. Cornell University, Ithaca, NY. 131 pp.
Boman, H.G. 1991. Antibacterial peptides: Key components needed in immunity. Cell 65:205-207.
Broekaert, W.F., Cammue, B.PA., De Bolle, M.F.C., Thevissen, K., De Samblanx, G.W., Osborn, R.W. 1997. Antimicrobial peptides from plants. Critical Reviews in Plant Sciences 16: 297-323.
Carlsson A., Nyström, T., de Cock, H., and Bennich, H.. 1998. Attacin-an insect immune protein-binds LPS and triggers the specific inhibition of bacterial outer-membrane protein synthesis. Microbiology 144:2179-2189.
Düring, K., Porsch, P., Fladung, M. and Lörz, H. 1993. Transgenic potato plants resistant to the phytopathogenic bacterium Erwinia carotovora. Plant J. 3:587-598.
Huang, W., Cui, X., Tian, Y., Lin, M., Peng, X. 1994. Cloning of T7 lysozyme gene and construction of the vector for transgenic plants resistant to bacterial infection. Weishengwu Xuebao 34: 261-265.
Huang, Y., and McBeath, J.H. 1997. Plant promoters controlled expression of cecropin B gene in transgenic potatoes renders disease resistance to soft rot bacteria. Phytopathology 87:S45. (Abstr.)
Hultmark, D., Engström, Å., Andersson, K., Steiner, H., Bennich, H., and Boman, H.G. 1983. Insect immunity. Attacins, a family of antibacterial proteins from Hyalophora cecropia. EMBO J. 2:571-576.
Jaynes, J. M., Xanthopoulos, K.G., Destéfano-Beltrán, L., and Dodds, J.H. 1987. Increasing bacterial disease resistance in plants utilizing antibacterial genes from insects. Bioassays 6:263-270.
Kato, A., Nakamura, S., Ibrahim, H., Matsumi, T., Tsumiyama, C., and Kato, M. 1998. Production of genetically modified lysozymes having extreme heat stability and antimicrobial activity against Gram negative bacteria in yeast and in plants. Nahrung 42: 128-130.
Ko, K. 1999. Attacin and T4 lysozyme transgenic 'Galaxy' apple: Regulation of transgene expression and plant resistance to fire blight (Erwinia amylovora). Ph.D dissertation, Cornell University, Ithaca, NY. 194 pp.
Ko, K., Norelli, J.L., Reynoird, J.-P., Boresjza-Wysocka, E., Brown, S.K., Aldwinckle, H.S. 2000. Effect of untranslated leader sequence of AMV RNA 4 and signal peptide of pathogenesis-related protein 1b on attacin gene expression, and resistance to fire blight in transgenic apple. Biotechnology Letters 22:373-381.
Lorito M., Woo, S.L., Fernandez, I.G., Colucci, G., Harman, G.E., Pintor-Toro, J.A., Filipponem, E., Muccifora, S., Lawrence, C.B., Zoina, A., Tuzun, S., and Scala, F. 1998. Genes from mycoparasitic fungi as a source for improving plant resistance to fungal pathogens. Proc. Natl. Acad. Sci. 95:7860-7865.
Mitra, A. and Zhang, Z.. 1994. Expression of a human lactoferrin cDNA in tobacco cells produces antibacterial protein(s). Plant Physiol. 106:977-981.
Nakajima H., Muranaka, T., Ishige, F., Akutsu, K., Oeda, K. 1997. Fungal and bacterial disease resistance in transgenic plants expressing human lysozyme. Plant Cell Reports 16: 674-679.
National Research Council. 1989. Alternative Agriculture: Committee on the Role of Alternative Farming Methods in Modern Production Agriculture, Board on Agriculture, National Academy Press, Washington, D.C.
Norelli, J.L., Aldwinckle, H.S., Destéfano-Beltrán, L., and Jaynes, J.M. 1994. Transgenic 'Malling 26' apple expressing the attacin E gene has increased resistance to Erwinia amylovora. Euphytica 77:123-128.
Norelli, J.L., Mills, J.Z., Momol, M.T., Aldwinkle, H.S. 1998. Effect of cercropin-type transgenes on fire blight resistanc of apple. Acta Horticulturae 489:273-278.
Norelli, J.L., Borejsza-Wysocka, E., Momol, M.T., Mills, J.Z., Grethel, A., Alkwinckle, H.S., Ko, K., Brown, S.K., Bauer, D.W., Beer, S.V., Abdul-Kader, A.M., Hanke, V. 1999. Genetic transformation for fire blight resistance in apple. Acta Horticulturae 489:295-296.
Reynoird, J.P., Mourgues, F., Norelli, J.L., Aldwinckle, H.S., Brisset, M.N., and Chevreau, E. 1999. First evidence for differences in fire blight resistance among transgenic pear clones expressing attacin gene. Plant Science 149:23-31.
Tronsomo, A. and Harman, G.E. 1993. Detection and quantification of N-acetyl-ß -D-glucosaminidase, chitobiosidase, and endochitinase in solutions and on gels. Anal. Bioechem. 208:74-79.
Trudel. J., Potvin, C., Asselin, A. 1995. Secreted hen lysozyme in transgenic tobacco: Recovery of bound enzyme and in vitro growth inhibition of plant pathogens. Plant Science 106: 55-62.
Vunnam, S., Juvvadi, P., and Merrifield, R.B. 1997. Synthesis and antibacterial action of cecropin and proline-arginine-rich peptides from pig intestine. J. Peptide Res. 49:59-66.
Wachinger, M., Kleinschmidtet, A., Winder, D., von Pechmann, N., Ludvigsen, A., Neumann, M., Holle, R., Salmons, B., Efle, V., and Brack-Werner, R. 1998. Antimicrobial peptides melittin and cecropin inhibit replication of human immunodeficiency virus 1 by suppressing viral gene expression. Journal of General Virology 79:731-740.
Wilson, C.R. and Conner, A.J. 1995. Activity of antimicrobial peptides against the causal agents of common scab, black leg and tuber soft rot diseases of potato. New Zealand Natural Sciences 22: 43-50.
Wu, G.S., Shortt, B.J , Lawrence, E.B , Leon J., Fitzsimmons, K.C., Levine, E.B., Raskin, I., Shah, D.M. 1997. Activation of host defense mechanisms by elevated production of H2O2 in transgenic plants. Plant Physiol., 115:427-435
RETURN TO APSnet FEATURE STORY