Ariel Castro
Dept. of Crop and Soil Sciences, Oregon State University and Dept. de Produccion Vegetal, Facultad de Agronomia, Universidad de la Republica, Uruguay
Castro, A. 2001. Cultivar Mixtures. The Plant Health Instructor. DOI: 10.1094/PHI-A-2001-1230-01.
Updated 2007.
Introduction
High crop yields are a principal objective of modern agriculture. Plant breeding has achieved high crop yields through hybridization and selection of superior plants. These superior types are often grown in monocultures where each plant is genetically identical to its neighbor. The genetic uniformity for plant height, maturity, and quality characteristics also facilitates harvesting, marketing, and processing of the crop. Wheat, maize, rice, potatoes, soybeans, and banana are some crops that are typically grown in monocultures.
Mixture of club wheat cultivars jackmar Tyree. (courtesy of C. Mundt)
|
A negative consequence of genetic uniformity is an increase in genetic vulnerability to disease caused by microbial pathogens. Plant diseases can prevent a crop from achieving its yield potential, and the cost of disease and its prevention can dramatically affect the economics of crop production.
If genetic uniformity makes a crop more vulnerable to disease, then one potential, low cost method of suppressing disease is to increase the genetic diversity of the crop. A simple way to enhance genetic diversity is to mix the seed of cultivars (i.e. plant genotypes) that vary in their susceptibility to specific pathogens. This method ensures genetic diversification with the advantage that it can be used in addition to any other form of disease control (Wolfe 1988).
This unit describes theoretical, experimental and practical results obtained using mixtures of crop cultivars for disease suppression. The information is targeted at students who have had or are currently enrolled in an upper division or graduate level class in plant pathology.
What is a cultivar mixture?
Wolfe (1985) defined cultivar mixtures as "mixtures of cultivars that vary for many characters including disease resistance, but have sufficient similarity to be grown together." Cultivar mixtures do not cause major changes to the agricultural system, generally increase yield stability, and in some cases can reduce pesticide use. They are also quicker and cheaper to formulate and modify than "multilines," which are defined as mixtures of genetically uniform lines of a crop species (near-isogenic lines) that differ only in a specific disease or pest resistance (Browning and Frey 1981).
Cultivars used in the mixture must possess good agronomic characteristics and may be phenotypically similar for important traits including maturity, height, quality and grain type, depending on the agronomic practices and intended use. Cultivar mixtures in barley for the control of powdery mildew are an example of phenotypically similar mixtures, whereas red- and white-grained sorghum mixtures used in Africa are an example of phenotypically different mixtures.
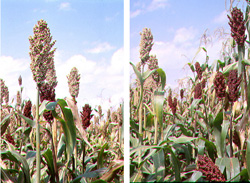 |
Mixtures of red-grained and white-grained sorghum (courtesy of Dr. Henry Ngugi, Pennsylvania State University) |
Another situation in which mixtures may be of economic interest is for the protection of susceptible host genotypes with superior agronomic characteristics. In that case, the deployment of the susceptible host in combination with an agronomically inferior, but disease resistant genotype, may be a solution (Garrett and Mundt 1999).
Mechanisms by which cultivar mixtures suppress disease
Cultivar mixtures do not completely suppress or eliminate the disease. Rather, mixtures reduce the rate of disease progress by eliminating large numbers of spores at each cycle of pathogen multiplication. Spores are eliminated from the epidemic process by deposition on resistant plants and by dilution because of the greater distance between plants of the same genotype. Moreover, the infection process may be slowed by the induction of defense responses in susceptible plants by strains of the pathogen that are avirulent on specific host genotypes. The result is a level of disease suppression owing to multiple epidemiological and physiological mechanisms. The four mechanisms by which cultivar mixtures suppress disease are summarized as follows:
a) Dilution effect. Increasing the distance between susceptible plants reduces/slows the rate of plant to plant spread.
b) Barrier effect. The presence of resistant plants in the canopy provides a physical barrier against spore dispersal, interrupting spore movement. The number and size of the resistant plants and the physics of spore dispersal influence the strength of the barrier effect
c) Induced resistance. Induced resistance occurs when biochemical host defenses are triggered by inoculation with an avirulent race. Triggering these defenses slows the infection processes of virulent pathogen races to which the host is normally susceptible.
d) Modification of the microclimate. The presence in the component cultivar of plant attributes (i.e. plant height, canopy traits, etc.) that modify the microclimate towards less favorable conditions for the disease can help the suppression of the disease.
In one pathogen generation, the combined effect of the four mechanisms in slowing the pathogen spread may be small. It is the multiplicative effect over several pathogen generations that leads to the greatest suppression of the disease (Wolfe 1985).
Dilution and barrier effects
A decrease in the density of susceptible plants slows disease development. If plants within the population possess different race-specific genes, the relative ability of any given pathogen race to spread from plant to plant is reduced because distance between plants of the same genotype is increased. For barley powdery mildew, Chin and Wolfe (1984) demonstrated that the increased distance between plants of the same genotype in cultivar mixtures was the most important mechanism of control, especially early in the epidemic. The ideal spatial arrangement of host genotypes is one in which plants susceptible to the same pathogen race do not occur as neighbors.
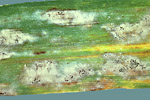 |
Cottony mycelial growth with dark, spherical cleistothecia of Blumeria graminis, the powdery mildew fungus, on barley. (Courtesy D. Mathre) Click image for an enlarged view. |
Similarly, the barrier effect is caused by the presence of a resistant plant that acts as a barrier to the spread of pathogen propagules (e.g., spores). For both of these mechanisms, the size of the host plant influences the effectiveness of the cultivar mixture. In general, mixture effectiveness decreases with increasing size of the host individuals (Garrett and Mundt 1999). The expected mixture effect for cereals, for example, is stronger than that expected for apples.
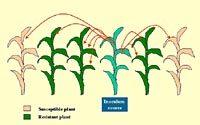 |
In a mixture, the number of released spores creating new infections can be reduced considerably by lowering the density of susceptible plants (dilution effect). Moreover, some of the spores released from an infected plant are captured by a resistant plant and consequently, removed from the epidemic process (barrier effect). Click image for an enlarged view. |
Induced resistance
Induced resistance occurs when spores of an avirulent strain or race land on and trigger a biochemical defense response on an incompatible host. This induction of defense responses reduces partially the susceptibility of the host plant to infection by spores of a virulent strain or race (Lannou et al. 1995). Either the infection efficacy or the number of new spores produced as a result of infection can be reduced (Martinelli et al. 1993) (see figure below). Induced resistance is a non-specific, general mechanism in many pathosystems and its characteristics vary from disease to disease. Some mechanisms of induced resistance are localized to tissues in the vicinity of an infection, but other mechanisms may affect a larger part of the plant. It has been suggested, however, that even very localized induction of resistance by an avirulent spore may result in significant disease reduction at the epidemic level (Lannou et al. 1995).
Experimental studies indicate that induced resistance may account for 20% to 40% of the disease reduction in mixtures when two or more pathogen races are active in the crop (Lannou and de Vallavieille-Pope 1997). According to Calonnec et al. (1996), up to one third of the reduction in infection by Puccinia striiformis in wheat mixtures was due to induced resistance. In this system induced resistance is particularly important because of the indeterminate (continually expanding) nature of stripe rust lesions. In barley powdery mildew, it is believed that induced resistance plays an important role during the latter stages of an epidemic (Chin and Wolfe 1984).
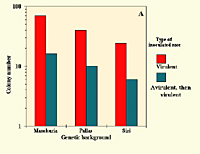 |
Numbers of colonies and spores of powdery mildew formed by Blumeria graminis f.sp.hordei on 2 cm x 5 cm barley leaf segments. Seedlings were inoculated either with an avirulent inducer isolate and then a virulent isolate, or with the virulent isolate only. Three sets of four near-isogenic lines, with different race-specific genes, were used. Values are the number of colonies per leaf segment (left) or number of spores per lesion (right) (from Martinelli et al. 1993). Click image for an enlarged view. |
Modification of the microclimate.
The presence in the component cultivar of plant attributes (i.e. plant height, canopy traits, etc.) that modify the microclimate towards less favorable conditions for the disease can help the suppression of the disease.
In a mixture of glutinous (35-40 cm taller and much more susceptible to rice blast) and non-glutinous rice, Zhu et al. (2005) have found that the interplanting of both types reduced the number of days with 100% humidity at 0800 h (from 20 for the pure stands to 2.2) and the mean percentage of glutinous leaf area covered by dew (from 84% to 36%). Both variables are critical for the development of the disease. This change in the environmental conditions was a substantial contributor to panicle blast control (over 90% reduction in incidence on the glutinous cultivar and 30-40% on the nonglutinous one), regardless of the effects of other mechanisms.
Effect of cultivar mixtures on epidemic development
The development of a plant disease epidemic is a function of the initial inoculum, the rate of disease development and the duration of crop growth (see APSnet Epidemiology topic). Cultivar mixtures affect the epidemic factors as follows:
a) The initial inoculum level can be reduced. Compared to a pure-line susceptible population, the initial inoculum is lowered when a given race or strain is not completely virulent on all genotypes in the mixture.
b) The rate of secondary infection can be reduced. As the proportion of susceptible tissue available for a given race of the pathogen is lowered, the number of new, secondary infections by this race is reduced, which results in a decrease in the observed 'apparent infection rate.'
Leonard's (1969) classic formula describes this second effect based on a single pathogen genotype in a simple mixture of one susceptible and one immune plant genotype:
X'/X0 = mn . X/X0
X = Proportion of infected tissue in a population composed only of the susceptible genotype
X'= Proportion of infected host tissue for the susceptible genotype in the mixture
X0= Proportion of host tissue initially infected
m = Proportion of susceptible plants in the mixture
n = Number of generations of successful infection and reproduction by the pathogen
Although this formula is a simplification of reality (for example, it doesn't consider the spatial configuration of host genotypes and the pattern of inoculum dispersal), it summarizes the 'mixture effect' as a function of m and n. It predicts that the disease suppressing effects of the mixture will increase linearly with an increasing proportion of resistant plants (lower m) but exponentially with more cycles of pathogen infection and reproduction (higher n). Thus, mixtures are most effective against pathogens with polycylic disease cycles (see APSnet Epidemiology topic).
Empirical results in maize (see figure below) show that mixtures of resistant and susceptible genotypes slow the rate of increase of common maize rust (measured as the cumulative number of pustules per susceptible plant) compared with pure stands of susceptible genotypes. The table at the bottom of this page shows other examples of cultivar mixtures in which some degree of disease suppression was achieved.
|
Cumulative numbers of common maize rust pustules on susceptible plants in pure stands and in mixtures of 25% susceptible and 75% resistant plants (adapted from Mundt and Leonard, 1986). Click image for an enlarged view. |
Examples of degree of disease suppression achieved through the use of cultivar mixtures. |
Plant |
Pathogen |
Reference |
Trait |
Disease reduction |
Maize |
Rust |
Mundt and Leonard, 1986 |
Pustules/plant |
50% |
Snap beans |
Bean rust |
Mundt and Leonard, 1986 |
AUDPC |
30-60% |
Wheat |
Stripe rust |
Mundt, 1994 |
Severity |
14-64% |
Wheat |
Leaf rust |
Mundt, 1994 |
Severity |
45% |
Barley |
Scald |
Mundt et al., 1994 |
Severity |
12% |
Barley |
Scald |
Newton et al., 1997 |
Severity |
11-50% |
Barley |
Powdery mildew |
Newton et al., 1997 |
Severity |
0-20% |
|
|
|
|
|
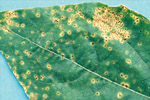 |
Rust, caused by Uromyces appendiculatus, on the upper leaf surface of a bean leaf. (Courtesy R. Hall) Click image for an enlarged view. |
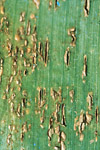 |
Uredinia of the common rust pathogen, Puccinia sorghi, on corn. (Courtesy C. De Leon) Click image for an enlarged view. |
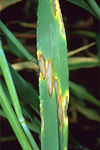
|
Typical leaf symptoms of barley scald. (Courtesy L. Jackson) Click image for an enlarged view. |
|
Cottony mycelial growth with dark, spherical cleistothecia of Blumeria graminis, the powdery mildew fungus, on barley. (Courtesy D. Mathre) Click image for an enlarged view. |
Effect of cultivar mixtures on the evolution of pathogen races or pathotypes
The effect of the cultivar mixtures on pathogen evolution can be analyzed based on two key questions:
i.) Will a given resistance be more durable when deployed in a mixture than deployed in a monoculture?
The lower level of exposure to the pathogen population of the resistance gene in a mixture compared with monoculture will reduce selection pressure on the pathogen population and therefore increase the gene's durability (Mundt, 2002).
ii) Considering a given number of resistance genes, will they be more durable if deployed in a mixture than deployed sequentially in monoculture or combined into a single host genotype?
Mixtures support more diverse pathogen populations than do pure stands, and that diversity is positively related to the degree of disease control provided by the mixture (Mundt, 2002). The mixtures lead, generally, to the evolution of complex pathogen races potentially capable of overcoming many resistance genes and therefore reduce the efficacy of the crop mixture as a measure to reduce disease. However, the appearance of complex races does not mean that those races will be selected for within the mixtures. There are several factors that can prevent the prevalence of complex races. The relative efficacy and durability of the resistance genes deployed in crop mixtures compared with the other two options, depends on the rate of progress of those races in the mixture, which can be affected by several factors:
- Diversity within pathotypes. Increasing diversity reduces the rate of increase of complex pathotypes in crop mixtures (Lannou, 2001)
- Fitness cost associated with virulence. The ability of complex races to attack multiple host genotypes is countered by a reduction in fitness associated with the lack of avirulence genes (Mundt and Lannou, 1997; Lannou, 2001; Mundt, 2002)
- Differential adaptation. Complex races can infect different host genotypes but have reduced infection efficiency compared with the specific simple races corresponding to each component of the mixture, reducing therefore their rate of progress (Lannou, 2001; Mundt, 2002).
- Density dependence. It is the decrease in pathogen multiplication rate associated with an increase in lesion density. It could affect simple and complex races differentially during an epidemic and reduce selection for complex races (Lannou and Mundt, 1997).
The progress towards complex pathogen races may be relatively slow. Single races or pathotypes capable of overcoming a single resistance gene should progress faster than complex races capable of overcoming multiple resistance genes, precluding the former of becoming dominant within the pathogen population.
Crops and diseases suited to cultivar mixtures
The effectiveness of cultivar mixtures has been demonstrated most commonly for foliar diseases of small grains in which host plants are small and for which there is frequent inoculum exchange among host genotypes. The effectiveness of cultivar mixtures in the control of foliar diseases of plants is related to the probability that pathogen propagules will fail to encounter susceptible tissues. Following Garrett and Mundt (1999) there are several inherent characteristics of each specific plant disease that affect this probability:
Size of genotype unit area (click here for more information)
Steepness of dispersal gradient (click here for more information)
Ultimate lesion size (click here for more information)
Pathogen generation time
Degree of host specialization (click here for more information)
Inherent characteristics of plant pathosystems that predict whether or not mixtures of cultivars will provide disease suppression. (from Garrett and Mundt, 1999). |
Host |
Pathogen |
Small host genotype unit area |
Shallow dispersal gradient |
Small lesion size |
Short pathogen generation time |
Strong host specialization |
Coffee |
Hemileia vastatrix |
- |
+ |
+ |
- |
+ |
Pepper |
Xanthomonas campestris |
- |
- |
- |
+ |
+ |
Potato |
Phytophthora infestans |
- |
+ |
- |
+ |
+ |
Rice |
Magnaporthe oryzae |
+ |
+ |
+ |
+ |
+ |
Wheat |
Blumeria graminis |
+ |
+ |
+ |
+ |
+ |
Wheat |
Puccinia triticina (formerly P. recondita) |
+ |
+ |
+ |
- |
+ |
Wheat |
Puccinia striiformis |
+ |
+ |
- |
- |
+ |
Wheat |
Mycosphaerella graminicola |
+ |
- |
- |
- |
- |
Wheat |
Rhizoctonia cerealis |
+ |
- |
- |
- |
- |
+ Characteristic favors disease suppression by cultivar mixtures - Characteristic does not favor disease suppression by cultivar mixtures. |
|
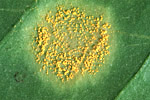
|
Uredinia of Hemileia vastatrix, causal agent of coffee rust. (Used by permission from J.R. Baker) Click image to enlarge.
|
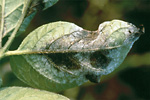
|
White sporangia and sporangiophores of Phytophthora infestans, causal agent of late blight, at the margins of necrotic potato leaf lesions. (Courtesy R.V. James) Click image to enlarge. |
|
Mature rice blast lesions showing necrotic borders caused by Magnaporthe grisea. (Courtesy J.M. Bonman) Click image to enlarge. |
Use of cultivar mixtures to manage multiple diseases
In field conditions crops can be attacked by multiple diseases, and cultivar mixtures can be used to manage and control those multiple diseases by combining cultivars with differential disease resistance to different diseases. The basic principles are the same as those used for controlling a single disease, with the addition of the possible interactions between the diseases present in the crop.
Experimental results show that cultivar mixtures can indeed control multiple diseases, and that the level of control is associated with the already mentioned characteristics of the specific plant disease (size of genotype unit area, dispersal gradient, lesion size, pathogen generation time and degree of host specialization).
Cox et al. (2004) studied the effect of wheat mixtures for the control of tan spot (caused by Pyrenophora tritici-repentis) and leaf rust (caused by Puccinia triticina). Mixtures consisted of two varieties, one resistant to tan spot and susceptible to leaf rust, and the other vice-versa. For both diseases, severity was lower on the susceptible cultivar in the mixture as compared with monoculture. The decrease was higher in leaf rust, which is a typical windborne pathogen, highly specialized, polycyclic, and with a shallow dispersal gradient. Tan spot, by contrast, is a residue-borne disease with low number of generations per growing season, capable of infecting several species, and with a steep dispersal gradient.
In another example, Ngugi et al. (2001) used mixtures of sorghum to control anthracnose (caused by Colletotrichum sublineolum) and leaf blight (caused by Exserohilum turcicum), considered the most destructive diseases of high yielding cultivars in eastern Africa. In this case they used one sorghum cultivar susceptible to both diseases and another with good resistance to both. Mixtures were effective in controlling both diseases, delaying the time when the disease was first observed and lowering the rate of disease progress. The effect was more pronounced with leaf blight, which is a wind-dispersed disease with shallow dispersal gradient and uniformly distributed incidence. Anthracnose is splash-dispersed, which is normally associated with steep gradients.
One important practical advantage of using cultivar mixtures to control multiple diseases is the relative ease of combining varieties with resistance to different diseases compared with the development of a single variety resistant to all diseases considered. Mixtures can therefore extend the options in deployment of host resistance.
How many cultivars make a good mixture?
The number of cultivars in the mixture can influence the disease control benefit achieved from it. Mundt (1994) showed that increasing the number of cultivars up to 5 gave a trend towards decreasing the severity of stripe rust on wheat (see table), but with potentially diminishing returns beyond 3 or 4 cultivar components. Newton et al. (1997), as shown in the figure below, obtained similar results in the control of scald on winter barley.
Percent reduction of stripe rust severity (relative to mean of component cultivars in pure stand) for wheat cultivar mixtures tested in field plots at three locations (from Mundt, 1994). |
Components in mixture |
Number of mixtures |
Meana |
2 |
10 |
31 |
3 |
10 |
42 |
4 |
5 |
49 |
5 |
1 |
48 |
Mean of all mixtures |
|
39 |
a Mean over three locations and 3-4 replications per location.
|
|
Change in scald severity in mixtures of winter barley cultivars compared as influenced by the number of component cultivars (adapted from Newton et al., 1997). Click image for an enlarged view. |
Reported successes with cultivar mixtures
Crop cultivar mixtures have been sown commercially in numerous countries with encouraging results:
Former GDR
From 1984 to 1990, cultivar mixtures comprised a substantial percentage (up to 92%) of the barley acreage of the former German Democratic Republic (East Germany). This country is the most remarkable example of large-scale use of this disease control strategy in industrialized agriculture.
China
A varietal diversification program was tested in the Yunnan province to control rice blast. Involving 812 ha in the first year and 3342 ha in the second year, mixtures of susceptible and resistant cultivars reduced the average rice blast severity on the susceptible varieties from 1% to 20% (Zhu et al. 2000). This experiment was unique in both its scale and experimental design.
USA
Wheat cultivar mixtures are increasingly popular in northeastern Oregon and southeastern Washington (Mundt 1994) for the objectives of stripe rust suppression and stabilization of yields. In 1998, 10% of soft white winter wheat and 76% of club wheat fields in this region were sown to cultivar mixtures. In Kansas, wheat variety blends occupied 7% of fields in 2000, with yield stabilization viewed as the most significant benefit received from mixture deployment (Bowden et al. 2001).
Switzerland
In 1992, a financial support program for cereal production ('Extenso') was introduced. Under 'Extenso' guidelines, applications of fungicides, insecticides and growth regulators are prohibited. As a consequence, the importance of cultivar mixtures for disease suppression has increased (Mertz and Valenghi 1997).
Denmark
In 1979, seed companies were allowed for the first time to produce and sell cultivar mixtures of spring barley. Winter barley mixtures with powdery mildew resistance were released in the mid-1980s. In 1996, 62,000 ha of barley (9.7% of the total) were sown to variety mixtures (Munck 1997). For the 1997 growing season, 49 different mixtures were marketed, involving 20 different varieties from six resistance groups to powdery mildew.
Poland
Use of barley cultivar mixtures for disease suppression was initiated in the early 1990's and has now reached about 90,000 ha per year (Gacek 1997). The main emphasis has been on spring-sown feed barley (eight mixtures recommended), but two spring-sown malting mixtures and three winter barley mixtures also have been recommended. The mixtures are designed for control of powdery mildew, but more general recommendations for their use are given to farmers. Before introduction into commercial production, candidate mixtures and their variety components are grown in field trials for three years at three or four sites. The best performing mixtures are selected for multiplication (initially as pure varieties with the final year as a mixture).
Colombia
Mixtures of coffee genotypes have been planted on a large scale in an effort to proactively reduce anticipated infection by Hemileia vastatrix in Colombia (Moreno Ruiz and Castillo Zapata, 1990).
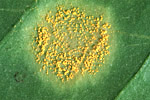 |
Uredinia of Hemileia vastatrix, causal agent of coffee rust. (Used by permission from J.R. Baker) Click image to enlarge. |
Agronomic considerations
With regard to cultivar mixtures, a basic question concerns whether increased genetic diversity among the individual crop plants is compatible with the production and marketing goals of the production system. Genotype and species mixtures are common in traditional agriculture. Current evidence also suggests that mixtures can work in commercial and modern agriculture (Mundt 1994, Bowden at al. 2001).
Mixtures have been frequently used for objectives other than disease control. Bowden et al. (2001) listed three advantages cultivar mixtures can provide: stabilization of yield (particularly when GxE, i.e. genotype by environment interaction effects account for a significant variation in yield), compensation effects (a strong variety compensates for a weak or injured variety) and disease control. Disease control may help to achieve the other two goals, but there also can be a direct effect of the mixture on yield stabilization and compensation.
Potential disadvantages of mixing cultivars also need to be considered. One practical disadvantage is the added time and cost involved in mixing. For example, some farmers lack the equipment to adequately mix seed. Incompatibility of the varietal components is another potential concern, especially with regard to plant height and maturity. This problem restricts the options for mixtures to components with similar heights and maturation times (Bowden et al. 2001). Another potentially important agronomic disadvantage of mixtures is the loss of the opportunity to adjust management practices to the specific requirements of each variety (e.g., plant density, fertilization, planting date, etc.)
Marketing restrictions and processing quality are often cited as major limitations to the use of mixtures. However, cultivars of the same market class are often bulked during handling and shipping. The German experience with barley demonstrated that adequate malting quality could be maintained in the face of widespread deployment of cultivars mixtures (Wolfe 1992).
Conclusion
For a particular host-pathogen system, it is very important to thoroughly test if mixtures can be useful for suppression of disease and stabilization of yield. Experimentation with simple mixtures of susceptible and resistant genotypes can be very helpful for testing whether the three basic mechanisms (dilution, barrier, and induced resistance) are active for that system (Garrett and Mundt 1999). The benefits achieved by cultivation of variety mixtures need to be carefully weighed against the agronomic considerations and requirements of the production system.
References
Bowden, R., J. Shoyer, K. Roozeboom, M. Claasen, P. Evans, B. Gordon, B. Heer, K. Janssen, J. Long, J. Martin, A. Schlegel, R. Sears, and M. Witt. 2001. Performance of wheat variety blends in Kansas. Kansas State University Agric. Extension Bull. 128 (www.oznet.ksu.edu/library/crpsl2/SRL128.pdf)
Browning, J. A., and K. J. Frey. 1981. The multiline concept in theory and practice. Pages 37-36 in: Strategies for the control of cereal disease, Jenkyn J. F., and Plumb, R. T., eds. Blackwell Scientific, London.
Calonnec, A., H. Goyeau, and C. de Vallavieille-Pope. 1996. Effects of induced resistance on infection efficiency and sporulation of Puccinia striiformis on seedlings in varietal mixtures and on field epidemics in pure stands. Euro. J. Plant Pathol. 102:733-741.
Chin, K. M., and M.S. Wolfe. 1984. The spread of Erysiphe graminis f.sp. hordei in mixtures of barley varieties. Plant Pathol. 33:89-100.
Cox C.M., K.A. Garret, R.L. Bowden, A.K. Fritz, S.P. Dendy, and W.F. Heer, 2004. Cultivar mixtures for the simultaneous management of multiple diseases: tan spot and leaf rust of wheat. Phytopathology 94: 961-969.
Fitt, B.D.L., and H.A. McCartney. 1986. Spore dispersal in relation to epidemic models. Pages 311-345 in: Plant disease Epidemiology, Volume 1, Leonard, K. J., and W.E. Fry, eds. McMillan, New York.
Gacek E., 1997. Summarized variety mixture information given to polish farmers. In: Variety Mixtures in theory and practice, Wolfe, M. S., ed. European Union Variety and Species Mixtures working group of COST Action 817. Online at: http://www.scri.ac.uk/
research/pp/pestanddisease/rhynchosporiumonbarley/otherwork/cropmixtures/
varietymixtures
Garrett, K. A., and C.C. Mundt. 1999. Epidemiology in mixed host populations. Phytopathology 89:984-990.
Jeger M.J., Griffiths E., and Jones D.G., 1981a. Disease progress of non-specialized fungal pathogens in intraspecific mixed stands of cereal cultivars. I. Models. Ann. Appl. Biol. 98: 197-198.
Jeger M.J., Griffiths E., and Jones D.G., 1981b. Disease progress of non-specialized fungal pathogens in intraspecific mixed stands of cereal cultivars. II. Field experiments. Ann. Appl. Biol. 98: 199-210.
Lannou C. 2001. Intrapathotype diversity for aggressiveness and pathogen evolution in cultivar mixtures. Phytopathology 91: 500-510.
Lannou C., C. de Vallavieille-Pope, C. Biass, and H. Goyeau. 1994. The efficacy of mixtures of susceptible
and resistant hosts in two wheat rusts of different lesion size: controlled condition experiments and
computerized simulations. J. Phytopathol. 140:227-237.
Lannou C., C. de Vallavieille-Pope, and H. Goyeau. 1995. Induced resistance in host-mixtures and its effect on disease control in computer-simulated epidemics. Plant Pathol. 44:478-489.
Lannou, C., and C. de Vallavieille-Pope. 1997. Mechanisms of variety mixtures for reducing epidemics. In: Variety Mixtures in theory and practice, Wolfe, M. S., ed. European Union Variety and Species Mixtures working group of COST Action 817. Online at: http://www.scri.ac.uk/research/pp/
pestanddisease/rhynchosporiumonbarley/otherwork/cropmixtures/varietymixtures
Lannou C., and C.C. Mundt. 1997. Evolution of a pathogen population in host mixtures: rate of emergence of complex races. Theor. Appl. Genet. 94: 991-999.
Leonard, K. L. 1969. Factors affecting rates of stem rust increase in mixed plantings of susceptible and resistant oat varieties. Phytopathology 59:1845-1850.
Martinelli, J. A., J. K. M. Brown, and M. S. Wolfe. 1993. Effects of barley genotype on induced resistance to powdery mildew. Plant Pathol. 42:195-202.
Merz, U., and D. Valenghi. 1997. "Extenso" production and cereal mixtures in Switzerland. In: Variety Mixtures in theory and practice, Wolfe, M. S., ed. European Union Variety and Species Mixtures working group of COST Action 817. Online at: http://www.scri.ac.uk/
research/pp/pestanddisease/rhynchosporiumonbarley/otherwork/cropmixtures/
varietymixtures
Moreno Ruiz G., and Castillo Zapata, J. 1990. The Variety Colombia: A variety of coffee with resistance to rust (Hemileia vastatrix Berk. And Br.). CENICAFE, Chinchina-Caldas, Colombia.Munk, L. 1997. Variety mixtures: 19 years of experience in Denmark. In: Variety Mixtures in theory and practice, Wolfe, M. S., ed. European Union Variety and Species Mixtures working group of COST Action 817. Online at: http://www.scri.ac.uk/research/pp/pestanddisease/
rhynchosporiumonbarley/otherwork/cropmixtures/varietymixtures
Mundt, C. C. 1989. Modeling disease increase in host mixtures. Pages 150-181 in: Plant Disease Epidemiology, Volume 2, Leonard, K. J., and Fry, W. E., eds. McGraw Hill, New York.
Mundt, C. C. 1994. Use of host genetic diversity to control cereal diseases: implications for rice blast. Pages 293-308 in: Rice Blast Disease, Zeigler, R. S., Leong, S. A., and Teng, P. S., eds. CAB International, London.
Mundt, C.C. 2002. Use of multiline cultivars and cultivar mixtures for disease management. Annu. Rev. Phytopathology 40: 381-410.
Mundt, C. C., and J. A. Browning. 1985. Development of crown rust epidemics in genetically diverse oat populations: effect of genotype unit area. Phytopathology 75:607-610.
Mundt, C. C., and K. J. Leonard. 1986. Effect of host genotype unit area on development of focal epidemics of bean rust and common maize rust in mixtures of resistance and susceptible plants. Phytopathology 76:590-598.
Mundt, C.C., P.M. Hayes, and C. C. Schon. 1994. Influence of barley mixtures on severity of scald and net blotch and on yield. Plant Pathology 43: 356-361.
Newton, A. C., R. P. Ellis, C. A. Hackett, and D.C. Guy. 1997. The effect of component number on Rynchosporium secalis infection and yield in mixtures of winter barley cultivars. Plant Pathol. 45:930-938.
Ngugi H.K., S.B. King, J. Holt, and M. Julian, 2001. Simultaneous temporal progress of sorghum anthracnose and leaf blight in crop mixtures with disparate patterns. Phytopathology 91: 720-729.
Wolfe, M. S. 1985. The current status and prospects of multiline cultivars and variety mixtures for disease control. Annu. Rev. Phytopathology 23:251-273.
Wolfe M.S., 1988. The use of variety mixtures to control disease and stabilize yield. Pages 91-100 in: Breeding strategies for resistance to the rust of wheat, Simmonds, N. W., and Rajaram, S., eds. CIMMYT, Mexico.
Wolfe M.S., 1992. Barley diseases: maintaining the value of our varieties. Pages 1055-1067 in: Barley Genetics Vol. VI, Munk, L., ed. Munksgaard International, Copenhagen.
Wolfe M.S., 1997. Variety mixtures: concept and value. In: Variety Mixtures in theory and practice, Wolfe, M. S. (ed.). European Union Variety and Species Mixtures working group of COST Action 817. Online at: http://www.scri.ac.uk/research/pp/
pestanddisease/rhynchosporiumonbarley/otherwork/cropmixtures/varietymixtures
Zhu, Y., H. Chen, J. Fan, Y. Wang, Y. Li, J. Chen, J. X. Fan, S. Yang, L. Hu, H. Leung, T. W. Mew, P. Teng, Z. Wang, and C. C. Mundt. 2000. Genetic diversity and disease control in rice. Nature 406: 718-722.
Zhu, Y., H. Fang, Y. Wang, J. Fang, S. Yang, T.W. Mew, and C.C. Mundt. 2005. Panicle blast and canopy moisture in rice cultivar mixtures. Phytopathology 95: 433-438.