Bacterial leaf streak is an important disease of wheat that can reduce yield up to 40% although losses are generally 10% or less. The disease is caused by
Xanthomonas translucens pv. undulosa and is widespread in areas where small grains are grown, especially in warm and humid climates or under sprinkler irrigation. This disease was first observed on barley in 1917 and later in wheat (1919) and rye (1924) and other grass species. The pathogen infects above ground parts of the plant including leaves, glumes, peduncles, and the rachis. Because the pathogen can infect glumes, seed-borne infections are a concern in the international exchange of germplasm.
Though reports of the disease are sporadic, bacterial leaf streak is distributed over a wide range of geographical areas. In North America, it has been reported in Canada, Mexico and the United States. In the United States, the disease has been most frequently reported from the lower mid-South region where warm, humid conditions favor disease, but recently the disease has become more prominent in Midwest wheat production areas. In South America, it occurs in Argentina, Bolivia, parts of Brazil, Paraguay, Peru and Uruguay. In Australia, it has been reported in New South Wales. In Asia, it is present in India, China, Pakistan and Iran. In addition, there are reports from the Middle East in durum and bread wheat in the irrigated areas of Syria, Turkey, and Yemen. In Africa, it has been reported in Kenya, Ethiopia, South Africa, Tanzania, Libya, Madagascar, Morocco and Zambia. To date, it has not been reported in Western Europe due to the relatively low temperatures in most wheat growing areas there, which are not favorable for the pathogen.
Initial leaf symptoms include water-soaked lesions extending along the leaf margins and midrib (Figure 2). When held up to incident light, lesions will appear translucent (Figure 3). Later these lesions may coalesce affecting larger areas of the leaf. Lesions will turn yellow in color and then eventually brown and necrotic (Figure 1). Under humid conditions, water-soaked lesions will produce honey-like exudates (sign of the pathogen) (Figure 2), and will harden into yellowish, resinous granules When there is moisture on the leaves, these honey-like exudates turn milky and later they spread on the leaf surface forming a thin layer of transparent flakes.
Bacterial propagules that land on the wheat spike (head) will cause black chaff. Symptoms include purplish-black longitudinal stripes on glumes that can also be seen on the peduncle and the rachis (Figure 4). Black chaff can result in seed transmission of the pathogen and in some cases can result in discolored kernels.
Figure 4. Symptoms of bacterial leaf streak on glumes and awns (Mary Burrows, Montana State University, Bugwood.org)
|
Pathogen Biology
Causal Organism
Xanthomonas translucens pv.
undulosa is a gram-negative non-spore forming, rod-shaped bacterium. Rods are 0.4 to 0.8 µm x 1.0 to 2.5 µm in size and appear either singly or in pairs. The bacterium has a single polar flagellum and forms long non-motile chains in peptonized nutrient broth augmented with 2% NaCl. The pathogen grows well at 28-30°C with growth slowing between 17-27°C and stopping at 36°C. The bacterial colonies produced on nutrient agar are round, yellow, mucoid, and shiny due to the production of the pigment xanthomonadin. The bacteria can be cultured readily on common media types like nutrient agar and Wilbrink's medium, which differentiates
X. translucens from many saprophytic bacteria. Yellow mucoid colonies on Wilbrink's media are characteristic of
X. translucens. Identification to the species level can be achieved by 16S rDNA sequencing. Pathogenicity tests are generally based on needle injections into the whorl of young plants or infiltrations of the flag leaf. After inoculation, plants are usually incubated for 24 hours at 25-30°C with 100% humidity followed by an additional 24 hours at ambient humidity, which aids in disease development. Virulent isolates produce water-soaked lesions as early as 3-4 days after inoculation (dai). Necrotic and chlorotic lesions usually appear within 7-10 dai.
Pathogen taxonomy
- Kingdom - Bacteria
- Phylum - Proteobacteria
- Class - Gammaproteobacteria
- Order - Xanthomonadales
- Family - Xanthomonadaceae
- Genus -
Xanthomonas
- Species -
Xanthomonas translucens
- Pathovar -
undulosa
The taxonomy of many species in the genus
Xanthomonas was resolved by Vauterin et al. (1995) by performing a polyphasic taxonomic study, which included a comprehensive DNA-DNA hybridization (DDH) comparison of 183 strains. The result was the description of twenty named species, with one of them being
X. translucens. Later Parkinson et al. (2007) reported a phylogeny of the diversity of
Xanthomonas species by comparing partial gyrase b gene sequences.
Xanthomonas translucens has since been confirmed as a separate species within the genus
Xanthomonas using both multilocus sequence analysis (MLSA) and whole genome sequencing Within the species
X. translucens, 10 named pathovars can be distinguished based on host range, symptoms, and phylogeny (Figure 5). Strains identified as
X. translucens pv.
undulosa isolated from wheat also can infect barley under certain circumstances, and strains identified as
X. translucens pv.
translucens isolated from barley also infect wheat, although rarely. However,
X. translucens pv.
undulosa strains usually are more virulent on wheat than on barley, while the opposite is true for strains of
X. translucens pv.
translucens. Moreover, while strains of the two pathovars are more closely related to each other than they are to other X. translucens pathovars, they are phylogenetically distinct from each other based on MLSA as well as whole genome sequencing. Combined with the observation that
X. translucens pv.
translucens strains and
X. translucens pv.
undulosa strains almost exclusively cause disease outbreaks on barley and wheat, respectively, there is strong etiological and epidemiological evidence that the barley and wheat pathovars, while related, are two different pathogens.
Figure 5. A. Phylogenetic tree of the genus Xanthomonas using MLSA. Nucleotide sequences of four housekeeping genes
(DnaK, gyrB, GroEL and
RecA) were extracted from the genomes. The scale bar indicates number of nucleotide substitutions per site. The dark red box represents strains of X. translucens pv. undulosa; the green box indicates strains of X. translucens pv.
translucens; the yellow box represents strains of X. translucens pv. cerealis;
B. Phylogenetic tree of
X. translucens strains using whole-genome discovery of Single Nucleotide Polymorphisms (Peng et al., 2016).
|
Additional information
Virulence Mechanisms
As for other species in the genus Xanthomonas, strains of
X. translucens pv.
undulosa have a Type II and Type III secretion system (T2SS and T3SS, respectively). Different plant cell degrading enzymes are secreted via the T2SS, such as cellulases, polygalacturonases, xylanases, and proteases. The T3SS is encoded by the
hrp/hrc (hypersensitive response & pathogenicity/hypersensitive response & conserved) locus and is used by the pathogen to inject so-called effector proteins into host cells, where they manipulate the host cell immune system and metabolism, creating an environment that is optimally suited for pathogen growth. The importance of the T3SS for pathogenicity of
X. translucens pv.
undulosa on wheat was recently shown. In fact, it was observed that the
hrc gene
hrcC is necessary for
X. translucens pv.
undulosa strain XT4699 to cause disease symptoms on the wheat cultivar 'Chinese Spring' upon inoculation, by either syringe infiltration or dip inoculation. The mutant also lost the ability to cause a hypersensitive response on the non-host tobacco. The effector repertoire of
X. translucens pv.
undulosa includes several transcription activator-like effectors (TALEs) which interact with promoter elements of host genes and thereby manipulate host gene expression.
Pathogen detection and diagnostics
Immunological assays. Several immunological methods have been developed to distinguish between different
X. translucens pathovars. Serological tests with specific antibodies can be used to confirm the presence of
Xanthomonas translucens pv.
undulosa. These types of assays generally require samples with high titers of bacteria or pure cultures. For example, rabbit polyclonal antibodies have been used for the detection of
Xanthomonas translucens pv.
undulosa in wheat seeds. Dot-immunobinding assays (DIA) are another type of serological test that can be used when the bacterial cell concentration is 105 cfu/ml or higher. DIA is a simple and cheap method but the threshold level for a positive reaction is high (105 cfu/ml). A different immunological technique is immunofluorescence, which is relatively quick and easy but more expensive than DIA. The sensitivity of this technique is higher than that of the DIA assay with a detection limit of 103-104 cfu/ml.
Nucleic acid-based identification - Currently, there is no molecular diagnostic test available to distinguish
X. translucens pv.
undulosa strains from other
X. translucens pathovars that cause disease on cereals, but a loop-mediated isothermal amplification (LAMP) assay has been developed to distinguish
X. translucens strains pathogenic on cereals from strains that are pathogens of non-cereals. The only molecular-based method to distinguish
X. translucens pv.
undulosa strains from other cereal pathovars is by multilocus sequence analysis or whole genome sequencing.
Disease Cycle and Epidemiology
Pathogen Survival and Dissemination
As BLS is seed borne, seed is the most common source of primary inoculum. In Idaho, more than 60% of seed lots were found to be contaminated, but epidemics did not occur unless more than 1,000 colony forming units (cfu) per gram were detected. From the seed surface, bacteria spread to the aerial parts of the plant and then infect the plumule through wounds and the coleoptile through stomata. After invading the coleoptile, infection of the foliage can occur prior to the emergence of the first leaf. Bacteria can also survive in the soil and crop debris. However, survival in air dried soil is limited to 14 days. Several grass species including canary grass (Phalaris canariensis L.), brome (Bromus inermis Leyss.), and timothy grass (Phleum pratense L.) serve as alternative hosts for the pathogen, but they contribute little to overwintering and long-term pathogen survival in the field.
Disease Cycle
Free water conditions (high relative humidity or rainfall) allow the pathogen to spread in the field. Bacteria enter the leaf interior through leaf stomata and multiply in the parenchyma thereby producing elongated longitudinal streaks which are limited by veins. Milky or yellow exudates are formed on the superficial lesions on the surface from which bacteria further spread by rain, wind and insects. Micro injuries caused by rain and/or wind can also lead to the penetration of bacteria into leaf blades (Figure 6).
Xanthomonas translucens pv. undulosa propagules can survive at a wide range of temperatures (15-30°C) but grow best above 26°C, and growth is severely reduced when relative humidity is less than 30%.
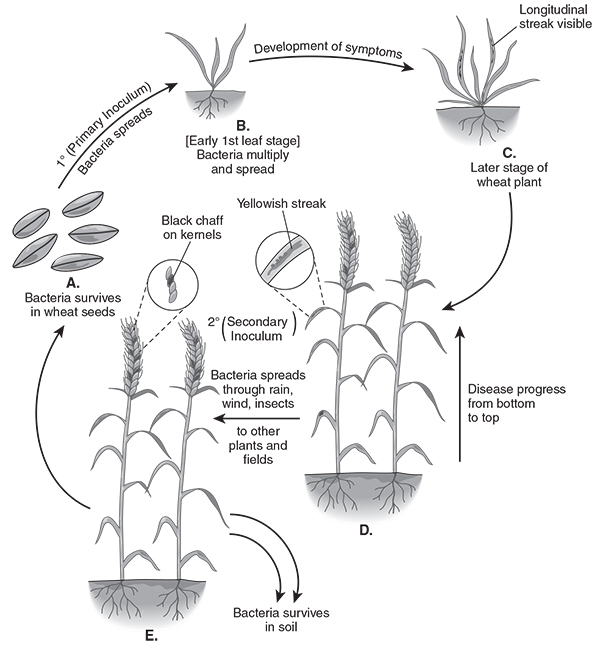 Figure 6. Disease cycle of Xanthomonas translucens pv. undulosa.
|
Disease Management
Cultural practices. Straw from a previously infected crop or weed hosts can serve as a source of overwintering inoculum, so field infestation can be reduced if the straw is incorporated into the soil. The pathogen is short-lived in soil, so excluding weed hosts and rotating to a non-host crop will contribute to reductions of inoculum in the field. However, since seed-borne inoculum is the most frequent source of primary infections, planting disease-free seed is critical for management of BLS.
Certified seed. Use of clean and pathogen-free seed is important, and seed multiplication should take place in disease-free areas under dry conditions without overhead irrigation. Tests can be conducted to determine the presence of the pathogen in the seeds (see section on pathogen detection above). For pathogen-free certification, a seed wash test on a semi-selective medium after dilution plating is widely used.
Chemical control. Seed treatments can reduce seedborne sources of inoculum, but they will not prevent outbreaks of BLS in the field if conditions are conducive for disease development. Seed treatment with acidified cupric acetate (0.5%) at 45°C for 20 minutes can reduce the incidence of BLS in the field due to seedborne infections. Studies have shown some reductions in disease with seed treatments including guazatine 300 g/L and imazalil 20 g/L, formaline, and dry heat, but these are not sufficient to prevent disease outbreaks when contaminated seed is planted.
Foliar-applied products with active ingredients based on mono- and dipotassium salts of phosphorous acid are labelled and commercially available for the control of BLS. These products, which are considered to have both fungicidal and bactericidal activity, are labelled for control of
Xanthomonas diseases in wheat. However, efficacy of these products for control of BLS has not been demonstrated, and they are not typically recommended for management of this disease.
Host resistance. Though commercial cultivars of wheat with high levels of BLS resistance are not available, some cultivars are less susceptible to the disease than others. Resistance to BLS is quantitative in nature and single gene resistance to BLS has not been identified. Major quantitative trait loci (QTL) associated with BLS resistance have been reported across the wheat genome from at least 9 of the 42 chromosomes (chromosomes 1A, 3A, 4A, 4B, 5A, 5D, 6B, and 7D). Wheat germplasm can be screened for resistance in either greenhouse assays or under field conditions. In greenhouse screening, seedlings and young plants can be evaluated by inoculating leaves at a concentration of 103 cfu/ml to determine the level of resistance that exists in different germplasm accessions. To promote infection and disease development following inoculation, plants are incubated in a humidity chamber (relative humidity >85%) for 5-7 days at 24-26 ºC.
For field screening, highly concentrated (109 cfu/ml) bacterial suspensions are sprayed onto plants at the tillering stage. Carborundum is added as an abrasive to facilitate entry of the pathogen into the plant. Disease incidence and disease severity can be measured at the flowering stage. Disease severity is assessed based on the percent leaf area with lesions (see Symptoms and Signs) and position of the lesions (i.e. height) within the plant canopy. By screening germplasm, additional QTLs associated with BLS resistance can be identified and used in breeding programs to select for wheat varieties with improved disease resistance.
Selected References
Adhikari, T. B., Gurung, S., Hansen, J. M., Jackson, E. W., and Bonman, J. M. 2012. Association Mapping of Quantitative Trait Loci in Spring Wheat Landraces Conferring Resistance to Bacterial Leaf Streak and Spot Blotch. The Plant Genome 5:1-16.
Bockus, W. W., Bowden, R., Hunger, R., Murray, T., and Smiley, R. 2010. Compendium of wheat diseases and pests. American Phytopathological Society (APS Press).
Bogdanove, A. J., Schornack, S., and Lahaye, T. 2010. TAL effectors: finding plant genes for disease and defense. Curr Opin Plant Biol 13:394-401.
Curland, R. D., Gao, L., Bull, C. T., Vinatzer, B. A., Dill-Macky, R., Van Eck, L., and Ishimaru, C. A. 2018. Genetic diversity and virulence of wheat and barley strains of
Xanthomonas translucens from the Upper Midwestern United States. Phytopathology 108:443-453.
Duveiller, E. 1994. A pictorial series of disease assessment keys for bacterial leaf streak of cereals. Plant Dis 78:137-141.
Duveiller, E., Fucikovsky, L., and Rudolph, K. The bacterial diseases of wheat concepts and methods of disease management/ed. by Etienne Duveriller, Leopold Fucikovsky and Klaus Rudolph. CIMMYT.
Duveiller, E., Bragard, C., Maraite, H., Curtis, B., Rajaram, S., and Gomez Macpherson, H. 2002. Bacterial leaf streak and black chaff. FAO Plant Production and Protection Series (FAO).
Hersemann, L., Wibberg, D., Blom, J., Goesmann, A., Widmer, F., Vorhölter, F.-J., and Kölliker, R. 2017. Comparative genomics of host adaptive traits in
Xanthomonas translucens pv.
graminis. BMC Genomics 18:35.
Jones, L., Johnson, A., and Reddy, C. 1916. Bacterial blights of barley and certain other cereals. Sci 44:432-433.
Kandel, Y. R., Glover, K. D., Tande, C. A., and Osborne, L. E. 2012a. Evaluation of spring wheat germplasm for resistance to bacterial leaf streak caused by
Xanthomonas campestris pv.
translucens. Plant Dis 96:1743-1748.
Kandel, Y. R., Glover, K. D., Tande, C. A., and Osborne, L. E. 2012b. Evaluation of spring wheat germplasm for resistance to bacterial leaf streak caused by
Xanthomonas campestris pv.
translucens. Plant Dis 96:1743-1748.
Langlois, P. A., Snelling, J., Hamilton, J. P., Bragard, C., Koebnik, R., Verdier, V., Triplett, L. R., Blom, J., Tisserat, N. A., and Leach, J. E. 2017. Characterization of the
Xanthomonas translucens complex using draft genomes, comparative genomics, phylogenetic analysis, and diagnostic LAMP assays. Phytopathology 107:519-527.
McMullen, M. P., and Adhikari, T. B. 2011. Bacterial leaf streak and black chaff of wheat. NDSU Extension Service, North Dakota State University.
Parkinson, N., Aritua, V., Heeney, J., Cowie, C., Bew, J., and Stead, D. 2007. Phylogenetic analysis of Xanthomonas species by comparison of partial gyrase B gene sequences. Int J Syst Evol Microbiol 57:2881-2887.
Peng, Z., Hu, Y., Xie, J., Potnis, N., Akhunova, A., Jones, J., Liu, Z., White, F. F., and Liu, S. 2016. Long read and single molecule DNA sequencing simplifies genome assembly and TAL effector gene analysis of
Xanthomonas translucens. BMC Genomics 17:21.
Ryan, R. P., Vorhölter, F.-J., Potnis, N., Jones, J. B., Van Sluys, M.-A., Bogdanove, A. J., and Dow, J. M. 2011. Pathogenomics of
Xanthomonas: understanding bacterium–plant interactions. Nature Reviews Microbiology 9:344.
Sharma, A., Sharma, D., and Verma, S. K. 2017. Proteome wide identification of iron binding proteins of
Xanthomonas translucens pv.
undulosa: focus on secretory virulent proteins. BioMetals 30:127-141.
Smith, E. F., Jones, L., and Reddy, C. 1919. The black chaff of wheat. Sci 50:48.
Vauterin, L., Hoste, B., Kersters, K., and Swings, J. 1995. Reclassification of
Xanthomonas. Int J Syst Evol Microbiol 45:472-489.
Young, J., Park, D.-C., Shearman, H., and Fargier, E. 2008. A multilocus sequence analysis of the genus Xanthomonas. Syst Appl Microbiol 31:366-377.