Parke, J. L., and E. K. Peterson. 2019. Sudden oak death, sudden larch death, and ramorum blight. The Plant Health Instructor. DOI: 10.1094/PHI-I-2019-0701-02
Sudden oak death, sudden larch death, ramorum leaf blight, ramorum shoot blight
Phytophthora ramorum
More than 150 species of forest trees, native shrubs, herbaceous plants, and woody ornamental plants
Authors
Jennifer L. Parke and
Ebba K. Peterson
Oregon State University, Corvallis, Oregon
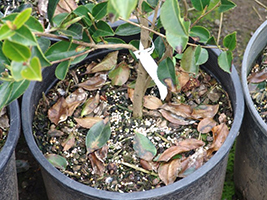 Sudden oak death of tanoak (left) and ramorum blight of camellia (right) caused by
Phytophthora ramorum. |
Phytophthora ramorum is a recently emerged pathogen with a host range of more than 150 plant species. This fungus-like organism causes sudden oak death on certain members of the oak family and has killed an estimated 30-45 million trees in coastal forests of California and Oregon. The pathogen additionally causes sudden larch death of Japanese larch, especially in conifer plantations in the United Kingdom. On most hosts, however,
P. ramorum causes ramorum leaf blight or shoot blight on native plant species and horticultural nursery crops, plaguing some nurseries in California, Oregon, Washington, British Columbia and Europe.
Symptoms and Signs
Phytophthora ramorum causes two distinct sets of symptoms, depending on the host species. On certain members of the Fagaceae (oak family) such as tanoak (Notholithocarpus densiflorus), and coast live oak (Quercus agrifolia) and other true oaks,
P. ramorum causes lethal bole (trunk) cankers resulting in the disease called sudden oak death (SOD). Lethal cankers are also observed on plantation Japanese larch (Larix kaempferi) as part of the disease sudden larch death (SLD). On a number of native plant species as well as many horticultural nursery crops,
P. ramorum causes a foliar blight and shoot dieback. The disease on these hosts is referred to as ramorum leaf blight or ramorum shoot blight.
Sudden oak death
Bole and crown symptoms
P. ramorum infects the phloem and inner bark to cause bleeding cankers on susceptible members of the Fagaceae. The presence of these bleeding cankers is the most diagnostic symptom of SOD. Initially, individual spots exhibit a clear, reddish exudate on the main trunk of the host (Figure 1). As the disease progresses, this exudate may continue to seep through both cracked and intact bark (Figure 2). The affected area eventually stains a dark reddish-brown as the bleeding is diffused by rainfall (Figure 3). Removal of the outer bark reveals discolored areas (cankers) in the inner bark, often surrounded by a black line (Figures 4, 5). Cankers range from a few centimeters to 3 meters in length, developing most often in the lower part of the trunk but also as high as 25 meters from the ground. For true oaks (Quercus spp.) cankers have only been found on mature trees, but they may appear on tanoak at any stage of development.
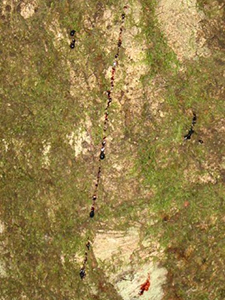 Figure 1. Bleeding of clear resin exudate
associated with early stages of symptom
development on the bole (trunk) of a tanoak.
| 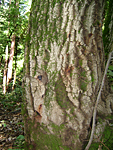 Figure 2. “Bleeding” symptom on older tanoak.
| 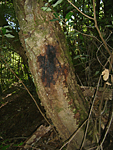 Figure 3. Extensive “bleeding” on severely
diseased tanoak, diffused by rainfall. |
P. ramorum may also infect sapwood, restricting water transport in xylem vessels. The crown of infected plants often appears off-color before individual branches or the entire crown turns brown and dies (Figure 6).
Foliar symptoms
Foliar symptoms are generally not observed on true oaks, but tanoak leaves in the canopy may exhibit mid-vein and petiole and twig necrosis. Infected stump sprouts, especially, may exhibit necrosis, stem lesions, and wilted shoot tips known as “shepherd's crooks" (Figures 7, 8).
Figure 7. Stump sprouts of tanoak infected with Phytophthora ramorum.
|
Figure 8. Foliar symptoms of sudden oak death on tanoak. The midrib and petioles are blackened and the leaf blade is necrotic. A young infected shoot shows a symptom known as a shepherd’s crook. |
Sudden larch death
Figure 9. Sudden larch death caused by P. ramorum. A) Death of mature trees in Northern Ireland; B) crown dieback; C) dead retained needles; D) bleeding lesion on stem; E) the maroon-colored under-bark. |
Sudden larch death (SLD) causes rapid decline and mortality of Japanese larch in European conifer plantations. The first symptoms may be crown dieback and premature browning of infected needles. Bleeding cankers are found on branches and main stems, most often located at the top of the tree, and lead to eventual death (Figure 9). Occasionally a stress crop of cones may be produced. Larch may be infected and die at any life stage.
European larch (L. decidua) and hybrid larches are also susceptible to SLD. In the United States, western larch (Larix occidentalis) is considered highly susceptible to
P. ramorum. However, its native range occurs well outside where environmental conditions are conducive for the establishment and spread of
P. ramorum, and it is not considered at risk for epidemic development.
Ramorum leaf blight and ramorum shoot blight
Most hosts of
P. ramorum exhibit symptoms chiefly on the foliage. On some hosts, lesions and vascular discoloration are associated with the stems. Plants susceptible to ramorum leaf blight or shoot blight (simply referred to henceforth as ramorum blight) include woody ornamental nursery stock as well as native herbs, shrubs, and trees other than the oak family hosts described above. On
Rhododendron, necrotic lesions may appear anywhere on leaf surfaces (Figure 10) and are easily confused with lesions caused by other leaf-infecting species of
Phytophthora. Leaf infection may occur through the petiole from infected stems, causing necrosis along the mid-vein (Figure 11). Foliar symptoms on other hosts vary, consisting of wedge-shaped or irregularly-shaped, darkened lesions that may appear watersoaked (Figure 12). Defoliation of affected camellia leaves is common (Figure 13). On California bay laurel (Umbellularia californica), necrosis is often confined to the leaf tip, with small yellow-brown spots scattered on other parts of the leaf (Figure 14). Conifers other than larch can also be hosts for
P. ramorum, although disease incidence is usually limited to young trees beneath heavily infected bay laurel or tanoak (Figure 15). For a description of symptoms on various hosts, refer to the following sources: Davidson et al., 2003; Rizzo et al., 2002; Tjosvold et al., 2005; and
The California Oak Mortality Task Force Website.
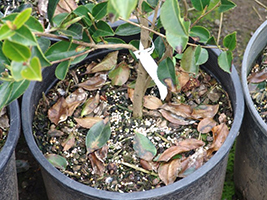 Figure 13. Camellia leaves with ramorum blight often fall to
the surface of the pot or onto the ground.
| 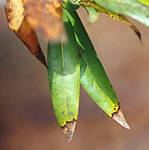
Figure 14. Leaf tip necrosis on California bay laurel.
Sporangia of
P. ramorum
are produced in
great abundance on this host during the rainy winters. Chlamydospores are also produced on the
leaves and may contribute to survival.
| 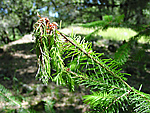
FIgure 15. A young Douglas-fir with symptoms of ramorum blight.
|
In greenhouse studies,
P. ramorum infects the roots of several hosts, although it does not produce root rot symptoms typical of soilborne
Phytophthora species. Root infection of nursery-propagated rhododendrons has been reported. The pathogen can spread from the roots and up the stem to above-ground plant parts. Root infection is often asymptomatic.
Signs
Although not detected on all hosts, sporangia (asexual fruiting structures) and hyphae of
P. ramorum can be found on leaves, usually on the lower surface (Figure 16). Sporangia are only formed under very moist conditions. Chlamydospores (asexually produced survival structures) may also be found on or in infected leaf tissue of foliar hosts (Figure 17), or in the wood and bark of canker hosts.
Figure 16. Sporangia of Phytophthora
ramorum produced on leaf tissue in vitro. |
Figure 17. Chlamydospores in a
rhododendron leaf. |
Pathogen Biology
Phytophthora ramorum is an oomycete (or water mold). No longer considered true fungi, these fungal-like organisms are now classified with brown algae in the Kingdom Stramenopila (or Straminipila, or Chromista).
P. ramorum was first isolated in Europe in 1993 but not described until 2001.
P. ramorum is placed in the family Peronosporaceae, order Peronosporales, and class Oomycota; molecular phylogeny shows it to be most closely related to
Phytophthora lateralis, a root pathogen of Port-Orford-cedar, and
P. hibernalis, which causes brown rot of citrus fruit.
P. ramorum has a unique set of morphological traits that can be observed on certain laboratory media (PARP medium, V8-juice agar, corn meal agar, or carrot agar). Vegetative growth consists of delicate, highly branched hyphae that grow in culture best at approximately 20°C (Figure 18). No mycelial growth is observed over 35°C. Asexual reproduction in the form of sporangia or chlamydospores is the most common form of reproduction for this oomycete. Sporangia can germinate directly or release motile zoospores. Large numbers of zoospores can be produced, ideally between the temperatures of 15-20°C. At 25°C, sporangia are more likely to germinate directly, by producing a germ tube. Sporangia are elongate, semi-papillate (have a short, rounded tip), and are sympodial (where each successive sporangium develops on a branch behind and to one side of the previous apex where growth has already ceased) (Figure 19). The sporangia of
P. ramorum are produced on a short (< 5 µm) to nonexistent pedicel (stalk), are caducous (fall off easily), and measure 25-97 x 14-34 µm (length x width).
P. ramorum sporangia are significantly more elongate than those of its closest relatives. This oomycete also forms many chlamydospores
in vitro, which are hyaline (clear) when young, and turn golden brown in older cultures (Figure 20). Chlamydospores of
P. ramorum are larger than chlamydospores of
P. lateralis, averaging 40-80 µm, and are mostly terminal and rarely lateral, although some can be intercalary (develop within the hyphal strand).
Figure 18. Phytophthora ramorum in culture
has a highly branched, delicate appearance. It produces sporangia and chlamydospores readily in vitro. |
Figure 19. Sporangia of Phytophthora ramorum, these with zoospores, are produced during wet weather. |
P. ramorum is heterothallic, requiring opposite mating types designated as A1 and A2, for sexual reproduction. The A1 mating type is most commonly found in Europe and was first described there. The A2 mating type is most common in the U.S., although the A1 type has been observed in North American nurseries since 2003 and in forests in Curry County, Oregon since 2015. Oospores (spores that result from sexual reproduction) are formed when an oogonium of one mating type is fertilized by the antheridium of the opposite mating type. Terminal oogonia are almost spherical and range in size from 24-40 µm in diameter (Figure 21). Antheridia are amphigynous (the oogonium grows through the antheridium) and rounded. Oospores have only been observed under laboratory conditions and their role in disease development in nature is not known.
Figure 20. Chlamydospores of P. ramorum are believed to be important in surviving the hot, dry summers.
|
Figure 21. Oogonium and antheridium formed by opposite mating types of Phytophthora ramorum in vitro. Sexual reproduction has not been observed outside the lab. |
Disease Cycle and Epidemiology
|
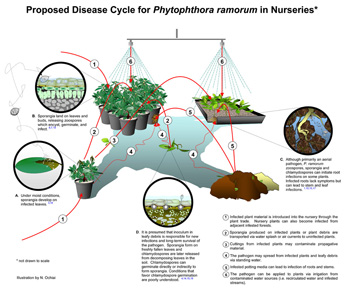 |
Disease cycle for
P. ramorum in California and Oregon forests (left; click to enlarge), and for
P. ramorum in the nursery (right; click to enlarge). Not shown: disease cycle for sudden larch death in the UK. |
Disease Cycle and Host Range
Because SOD, SLD and ramorum blight are diseases that have emerged relatively recently, many details of the disease cycles are not yet understood. It appears that
P. ramorum shares many characteristics of other aerially dispersed
Phytophthora species. After infection of the host tissue, sporangia are produced and dispersed to new host material. There, the sporangia either germinate or release motile zoospores. A new infection event occurs, and the asexual cycle is repeated.
Ramorum blight is a polycyclic disease (has more than one disease cycle per growing season) on foliar hosts, producing many sporangia during wet conditions. Chlamydospores are produced in abundance by this pathogen and likely serve as a reservoir of inoculum during conditions unfavorable for disease development. Most SOD hosts, such as coast live oak, are often considered "dead end" hosts because no sporangia are produced, and mortality occurs as a result of canker formation or colonization of sapwood. Many other hosts, such as California bay laurel, produce sporangia but do not suffer significant damage from the disease. Sporulation has been observed on leaves and petioles of only two canker hosts, tanoak and larch. Sexual reproduction has not been observed in nature.
P. ramorum has a large host range that includes more than 150 species. APHIS (the USDA Animal and Plant Health Inspection Service)
maintains a list of proven hosts and associated plants (associated plants are those from which
P. ramorum has been isolated but Koch's postulates have not yet been confirmed) for
P. ramorum. Between 2013-2018,
P. ramorum was reported on 20 additional plant species. Not all species in a particular genus are susceptible, and within a given species a gradient of susceptibility among varieties and individuals is often evident. The amount of disease observed in laboratory trials depends heavily upon the lineage, isolate and temperatures used in the virulence assay. Other factors may also cause differing responses by closely related species or cultivars.
Figure 22. Distribution of sudden oak death as of 2017 |
Genotyping of
P. ramorum isolates shows that there are four clonal lineages: EU1, EU2, NA1 and NA2 (Table 1). The EU1 and EU2 lineages, and the NA1 and NA2 lineages were first described in Europe and North America, respectively. Except for a single outbreak of the EU1 lineage in Oregon, forest epidemics in North America are exclusively of the NA1 lineage. NA2 has only been found in Pacific Northwest nurseries and surrounding waterways. EU2 was not described until 2012 and is thus far restricted to Northern Ireland and Scotland. The finding of both EU1 (mating type A1) and NA1 (mating type A2) isolates in a few nurseries and parts of Oregon raises the risk that sexual reproduction could occur. However, sexual reproduction has not yet been observed in these settings. Sexual reproduction for
P. ramorum may not be fully functional: gametangial formation is generally sparse and meiosis often occurs abnormally; genetic analysis has also determined the NA1, NA2, and EU1 lineages may be reproductively isolated in their center of origin.
Table 1. The lineages of Phytophthora ramorum in relation to distribution and mating type. The EU1, EU2, NA1, and NA2 lineages are clonal.
Lineage |
Current distribution |
Habitat |
Mating type |
EU1 | Europe United States | Nurseries, forests
| A1 |
EU2
| Europe
| Forests
| A1
|
NA1
| United States
| Nurseries, forests
| A2 |
NA2
| United States
| Nurseries
| A2
|
undescribed
| Vietnam
| Forests
| A1 and A2
|
Because
P. ramorum does not occur throughout the range of susceptible hosts and epidemics have limited genetic diversity, it is believed to be exotic to both North America and Europe. Much effort has been invested in discovering this pathogen's native range. Subsequent surveys conducted in 2016 in Vietnam recovered both the A1 and A2 mating types from natural forests, supporting a Southeast Asian origin.
Dispersal
As with other aerial
Phytophthoras, P. ramorum is likely transmitted in forests by rain splash, air currents and wind-driven rain, but many specific details of dispersal and infection are not fully known. There is a high risk of propagule transmission by humans who move infested plant material and soil, as observed for closely related
Phytophthora species. Viable propagules can get lodged on the shoes of hikers or on bicycle tires. And while these propagules may be transmitted to new locations, limited evidence supports this as the dominant dispersal mechanism in forests. Rather, sporangia are believed to be moved by rain splash and air currents following wet weather. They likely initiate leaf and twig infections high in the forest canopy. Following rain or fog, more sporangia are produced, which can be blown or splashed to other leaves or to the boles of canker hosts.
P. ramorum can also be recovered from streams in infested areas, but it does not appear that this has led to new infections in the forest. While other potential bird, mammal, and insect vectors have had limited assessments of their ability to transmit
P. ramorum, all negative, the pattern of disease occurrence in the forest is most consistent with windborne dispersal of inoculum.
In the forest setting,
P. ramorum follows a classic dispersal gradient whereby the number of propagules decreases with increasing distance from site of formation. Most inoculum remains within 10 m of the host. Yet, it is possible that a few propagules are dispersed long distances. Epidemic development has been best mapped in Curry County, OR. Here, the vast majority of new sites resulting from aerially dispersed inoculum occur within 600 m of the nearest known source of inoculum; maximum dispersal distances may be as far as 8 km during rare storm events.
In horticultural nurseries, infections have been found up to 0.5 m from the closest infected plant, but infections due to long distance wind dispersal in this environment have not yet been noted. Rather, the majority of spread within a nursery appears to occur between adjacent, touching plants and exposure to infested surface water. Irrigation water can be an inoculum source for nurseries using contaminated recycled irrigation water or water from infested streams. It is also likely that plants may occasionally become infected from inoculum reserves in the soil. Rhododendrons and some other nursery plants can become infected through their roots if inoculum is present in the potting mix.
Long-distance dispersal of
P. ramorum is facilitated by shipments of infected nursery plants, which is the most likely mechanism for introduction into Europe and North America. It is likely that at least five separate intercontinental migration events have occurred (Figure 23).
P. ramorum was first detected in California nurseries in 2001. In 2004, a large wholesale nursery in southern California shipped 1.6 million camellias throughout the U.S. (Figure 24, 25). The nursery was later found to be infested with
P. ramorum (Figure 26). Although APHIS checked each of the nurseries that had received plants and destroyed the plants that were infected, many had already been sold. Despite fears that these infected camellias might spread the disease to surrounding forests, and the detection of
P. ramorum from waterways associated with previously infested nurseries, there is no evidence yet that
P. ramorum has become established in forests outside of California and Oregon. Shipment of
P. ramorum by some nurseries continues to be a problem.
Figure 23. Scenarios depicting five intercontinental migrations of Phytophthora ramorum based on population genetic and evolutionary studies (Grünwald et al. 2012; Van Poucke et al. 2012). |
Figure 24. Young camellia liners in this nursery were infected with Phytophthora ramorum.
|
Figure 25. Young camellia liners in this nursery were infected with Phytophthora ramorum.
|
Figure 26. Map showing locations (open circles) where potentially infected nursery plants were shipped from a California nursery in 2004. Sites where Phytophthora ramorum was detected are indicated by blue stars. |
Epidemiology
The precise epidemiology of
P. ramorum differs upon the location and hosts present. In general, locations with mild winters and heavy rainfall favor epidemic development. Wetter years, particularly those with greater precipitation during the warmer spring months, are associated with greater disease development. Increased foliar symptoms are often readily apparent the year of and year following such events. Subsequent increases in mortality tend to occur 1-3 years after favorable conditions due to the delay between initial infection and mortality. Due to irrigation and other factors, nursery epidemics may occur in areas with much colder winters and warmer summers than would be considered optimal for disease development.
P. ramorum does not sporulate on all hosts. With the exception of larch and tanoak, sporangia are not produced from the bole hosts that succumb to
P. ramorum. Rather, sporangia are readily produced on foliar hosts, which are then spread by rain splash to infect adjacent bole hosts. In the U.K. the dominant sporulating host (prior to the development of SLD) is rhododendron. In California the dominant sporulating host is California bay laurel (Umbellularia californica), which is commonly found in association with oaks in infested forests. Ramorum blight is not lethal on bay laurel or any other strictly foliar host. Therefore,
P. ramorum can persist in infested forests on many native hosts in the absence of oak.
Larch and tanoak are the two hosts that both produce abundant sporangia and can be killed by SLD and SOD. Sporulation from infected larch needles is especially abundant, and is driving the development and spread of SLD in the U.K. In Oregon,
P. ramorum appears to be predominantly spread by tanoak, despite the abundance of bay laurel in many heavily infested areas.
By and large the epidemiology of
P. ramorum has focused on the production and spread of sporangia, though chlamydospores are also produced in abundance in some hosts. The ecology of chlamydospores is poorly understood. When infested leaves fall to the ground or chlamydospores are shed, it is hypothesized they may lie dormant during the dry summer, then eventually germinate and produce more sporangia when the winter rains arrive, initiating new infections.
P. ramorum can survive in buried, infested leaf debris for at least 33 months, which is attributed to formation of chlamydospores. Soil inoculum sources are likely more important in the initiation of epidemics in nurseries than in forests. While the infection of above-ground foliage from soil sources has been demonstrated in forests, multiple lines of evidence have indicated that inoculum produced in tanoak, bay laurel, or larch canopies is responsible for most new infections in forest habitats.
Disease Management
Because
Phytophthora ramorum is a quarantined pathogen that affects plants in both forests and nurseries, disease management is complex. It requires cooperation among federal, state, and county agencies as well as private industry to protect natural resources while not unduly limiting forest and nursery commerce.
Diagnosis and Monitoring
It is important to quickly and accurately identify symptoms caused by
P. ramorum in the nursery, forest, or landscape. Since symptoms vary by host and are not diagnostic, best results have been achieved by combining polymerase chain reaction (PCR) based assays with the more traditional method of isolating the pathogen from symptomatic tissue on the selective laboratory medium PARPH. PCR primers specific for
P. ramorum are available. Enzyme-linked immunosorbent assay (ELISA) kits designed for field detection of
Phytophthora can be a useful screening tool, though they cannot determine which species are present.
Nursery personnel should systematically inspect and test plants and propagative material to prevent introduction of the pathogen to existing stock. In the field, monitoring must continue in both infested and non-infested areas. The use of aerial and ground surveys, stream baiting in high-risk watersheds, and monitoring by trained members of the community must all be used in concert. Effective education and communication are essential for preventing the spread of
P. ramorum. Programs and training sessions have been established for nursery employees, natural resource managers, Master Gardeners, Extension personnel, arborists, landscapers, utility workers, recreationalists and homeowners. Several on-going citizen science initiatives engage the public in the collection of plant or stream samples for
P. ramorum testing. A free mobile app, SODmap, is also available. It shows the distribution of SOD-positive and SOD-negative trees based on laboratory results. Web-based resources include photos of symptoms on hosts and recommendations for disease management.
APHIS provides funding to states for nursery surveys of host plants. The U.S. Forest Service with state cooperators conduct stream surveys for early detection of
P. ramorum outside the quarantine areas. Among several states (AL, GA, MS, NC, PA, SC, TX, WA) participating in the 2018 survey,
P. ramorum was detected in AL, MS, NC, and WA, in streams near nurseries previously found positive for
P. ramorum. Even though
P. ramorum has been consistently detected in some of these streams for years, there is no evidence the pathogen has initiated disease from waterborne inoculum, or that the pathogen has established in forests outside the quarantine areas in California and Oregon.
Exclusion
The most effective way to prevent
P. ramorum from becoming established is to keep it out of non-infested areas. Preventing human-facilitated spread of the disease is critical. Federal and state quarantines were implemented to prevent the spread of
P. ramorum with infected nursery plants. Initially, a federal order required that all west coast nurseries that shipped hosts interstate be inspected, tested, and certified as free from
P. ramorum. In 2015, steps were taken to deregulate the lowest risk areas and nurseries and to concentrate on highest risk sources for interstate dissemination of the pathogen. Nurseries that have previously been positive for
P. ramorum and which ship
P. ramorum hosts out-of-state now must enter into a federal compliance agreement that requires twice yearly inspection and sampling for
P. ramorum. Nurseries that participate in the compliance agreement must remain free of
P. ramorum for three years. All other nurseries that ship hosts intrastate, or ship non-hosts only, are inspected annually. Despite quarantines,
P. ramorum continues to be detected in some nurseries. In 2018, 11 California nurseries and 10 Oregon nurseries were positive for
P. ramorum. All were required to undergo the USDA Confirmed Nursery Protocol to delimit and eradicate the pathogen.
Specific “best management practices" (BMPs) are recommended to prevent establishment and further spread of the disease. BMPs include monitoring all known hosts for symptom development and inspecting all incoming plant stock for symptoms of disease. For a list of BMPs, refer to the
California Oak Mortality Task Force Website. Movement of infected logs (including firewood), infested soil, and litter outside infested counties are all strictly prohibited. Even within quarantined counties, one should not move infested materials. To prevent further spread through California forests, signage and devices for cleaning shoes and bicycles have been installed along trailheads in some infested areas.
Avoidance
As part of BMP strategies to avoid introduction of
P. ramorum, growers are encouraged to segregate the five highest-risk genera (rhododendron, camellia, viburnum, kalmia, and pieris) from the rest of their nursery stock. They are also asked to avoid conditions that are conducive to the development of
Phytophthora diseases by improving drainage and irrigating in such a way as to minimize the period of leaf wetness. Nurseries in infested areas should also remove potential native hosts, especially bay laurel trees, from their property. A systems approach is recommended to identify potential sources of pathogen contamination and to target unsafe management practices.
Eradication
Eradication may be a viable option if the disease is caught very soon after introduction. For example, the state of Oregon established a proactive eradication program in 2001 in an attempt to control
P. ramorum in Curry County. Detection of new mortality is done through aerial surveys identifying recently dead, overstory tanoak. According to eradication protocols, all hosts within a minimum of 300 feet (approximately 90 m) of symptomatic hosts are cut, piled, and burned. Cut stumps are also treated with herbicides, when allowed, to reduce sprouting.
Following a sharp increase in the number of new sites detected in 2011, largely a result of leaving many infestations untreated the prior year, the program shifted from total eradication to slowing the spread. In 2012, quarantine regulations were changed to create a zone known as the Generally Infested Area (GIA) in which eradication was no longer required by law. Then in 2015 the EU1 lineage was found infecting tanoak. This was likely introduced from a nearby nursery, in which EU1 had been detected via routine
P. ramorum monitoring in 2012. Treatment priorities have since focused on detections nearer the quarantine boundary and any new EU1 detections. As of 2017 approximately 2,165 ha (5,350 acres) in Curry County have been treated (Figure 27).
This type of eradication program was only feasible because at the time of detection
P. ramorum was not already widespread, a mechanism of early detection was in place, and there was significant landowner cooperation. Although the pathogen has spread to new sites in Curry County, the rate at which
P. ramorum has moved north and intensified appears to be less than in similar forests in northern California where eradication programs were not implemented. There have also been instances in which new sites and pathogen introductions have been successfully eradicated prior to their local intensification.
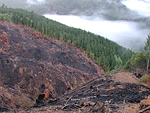
Figure 27. An eradication site in southwest Oregon.
All vegetation within 100 feet of infected trees was
cleared, piled, and broadcast burned in an attempt to
rid the site of Phytophthora ramorum. The site is then
monitored for 2 years to determine if the treatment
was effective. |
Figure 28. Individual nurseries affected by ramorum
blight have lost millions of dollars due to crop destruction
and lost sales. |
In all nurseries, infected plants must be destroyed as part of the eradication program. If infected plants are found in a particular nursery, corresponding nurseries receiving or sending these plant materials are also inspected as part of the APHIS protocol. Infected material at these sites, as well as plants within a buffer zone of 2 m, are then destroyed (Figure 28). Healthy-appearing plants within 4 m of infected plants are held for observation for a period of time to see if symptoms develop.
On a smaller scale, eradication of inoculum from soil or contaminated containers can be achieved through treatment with pasteurization (65°C for 30 min), steaming, solarization, or by treatment with soil fumigants. Biological control of soilborne inoculum of
P. ramorum with
Trichoderma asperellum has been demonstrated under certain circumstances. Two facilities in California are also certified to “hot compost" green waste that is infested with
P. ramorum.
Protection
Protection consists of treating a healthy plant before it comes in contact with the pathogen. An important aspect of fungicide application in nurseries is to ensure that treated plants are not already infected with the pathogen. Most Oomycete-specific fungicides do not kill
Phytophthora; rather they temporarily suppress symptom development, delaying detection until after the plants are shipped and the compounds are no longer applied. Therefore, if fungicides are used, they should only be used to prevent infection and should not be applied to potentially infected plants. Film-forming polymers and surfactants may be useful on plants being held for observation as they reduce sporulation without interfering with symptom expression. Several chemicals, including mefenoxam, dimethomorph, pyraclostrobin, fenamidone and oxathiapiproline, may protect nursery hosts such as rhododendron from infection. Permitted uses vary for different hosts and in different states and countries. While fungicide resistance has not yet been reported in the U.S., resistance of
P. ramorum to metalaxyl has been reported in Europe. There is still more work to be done to determine dosages, application timing, and hosts to be treated. Preventive treatment of forest trees with fungicides is not economically feasible, nor is it permitted on public lands. Individual high-value trees on private property may be treated with phosphite fungicides by direct injection or by mixing them with organosilicate surfactant-penetrants applied to the bark. Both methods have been shown to reduce disease development on canker hosts, and these chemicals are registered in California.
Resistance
There is considerable variation in host resistance among genera and species of susceptible plants. Resistant coast live oak trees have been observed in nature. Much less resistance has been observed for tanoak, although efforts to screen individuals and collect acorns from living trees in heavily infested areas are underway. Of the many plants that have been tested for susceptibility to
P. ramorum in laboratory studies, very few species appear to be immune. Variation in virulence has also been seen among U.S. isolates of
P. ramorum. So far, host specificity has not been found for particular isolates.
Significance
Other exotic
Phytophthora species have led to extensive tree mortality and negative ecological impacts in Australia, New Zealand, Europe, and North and South America. The desire to prevent an outbreak as devastating as historical epidemics like Dutch elm disease and chestnut blight has led to cooperative efforts between governmental officials, industry, academia, and the public.
Phytophthora ramorum is a recently emerged pathogen, thus the ultimate significance of SOD, SLD, and ramorum blight is still unknown.
The climate in coastal areas of Washington, Oregon, and California as well as large areas of the southeastern U.S. is considered suitable for
P. ramorum (Figure 29). In greenhouse tests, several important east coast tree species were found to be highly susceptible to the disease. The fear is this disease could spread by infested nursery plants to the eastern U.S. where susceptible oak-hickory, oak-pine, and lowland oak forests account for 67.8 million ha (167.5 million acres), or 46% of the total area classified as timberland. Still, despite repeated detections of
P. ramorum in nurseries and associated waterways in eastern states,
P. ramorum has not been found infecting native vegetation there. Models predicting climate suitability in the east vary significantly depending upon the methodology and model parameters; the extent to which the precise conditions required for epidemic establishment are met in the east requires further investigation.
Figure 29. Relative risk of
Phytophthora ramorum establishment based
on infection frequency, extreme cold temperatures, and host range.
Maps are based on 10 years of weather data (Magarey et al. 2007). |
SOD has already changed the landscape of coastal California and southwestern Oregon forests, where it is estimated that 30-45 million trees were killed by 2014. The disease is lethal to multiple oak species and tanoaks that make up a substantial part of several mixed-evergreen forest types. The loss of these species could affect wildlife and ecosystem processes in multiple ways: the loss of acorn-producing species endangers already threatened species such as the northern spotted owl; rapid mortality and changing community structure affects microclimate and nutrient cycling; mortality also dramatically increases erosion potential and fire risk.
The impacts SOD has on fire behavior depends upon the stage of the invasion; whereby greatest risk is apparent soon after
P. ramorum has entered an area. Dead crowns are especially flammable, and flushes of mortality contribute to a buildup of surface fuels. These impacts have been best studied for the 2008 Basin Complex & Chalk Fires and the 2016 Soberanes Fire in Big Sur (Monterey County, CA), and the 2017 Chetco Bar Fire in Curry County, OR. In no case did the fire eliminate
P. ramorum from the stand or from the soil. Rather, resprouting tanoak and bay laurel were commonly found infected even in heavily burned areas.
P. ramorum has also placed a financial burden on the nursery and forest industry on the west coast. Nursery sales make up a substantial portion of the agricultural economy for all three west coast states, and ramorum blight affects some of the most profitable and popular nursery crops for interstate commerce. Crop destruction, loss of sales, new disease management practices, inspections, training, testing, and increased documentation have required a huge commitment of both time and money.
P. ramorum has forced many changes in the nursery industry nationally, leading to greater awareness about
Phytophthora diseases generally and highlighting the need for more effective preventative methods to deal with quarantine pests and pathogens.
Douglas-fir is a major forest product in the northern portion of
P. ramorum's predicted range. As a host, the movement of Douglas-fir is regulated when produced in quarantine areas. Greater risk to the production and regulation of Douglas-fir has resulted from the establishment of the EU1 lineage in Oregon. While Douglas-fir foliage and branch tips have been found naturally infected when growing near heavily infested tanoak, no NA1 epidemic has occurred on Douglas-fir or any other conifer host in the United States. However, given EU1's capacity to cause substantial disease on larch, and its generally greater aggressiveness in laboratory trials, there is grave concern about the long-term outcome of an EU1 epidemic in conifer production regions.
Selected References
Brasier C., and Webber J. 2010. Sudden larch death. Nature 466:824-825.
California Oak Mortality Task Force.
http://www.suddenoakdeath.org/
Cobb R.C., R.K. Meentemeyer, and D.M. Rizzo. 2010. Apparent competition in canopy trees determined by pathogen transmission rather than susceptibility. Ecology 91(2):327-333.
Davidson J.M., H.A. Patterson, and D.M. Rizzo. 2008. Sources of inoculum for
Phytophthora ramorum in a redwood forest. Phytopathology 98:860-866.
Davidson J.M., H.A. Patterson, A.C. Wickland, E.J. Fichtner, and D.M. Rizzo. 2011. Forest type influences transmission of
Phytophthora ramorum in California oak woodlands. Phytopathology 101:492-501.
Davidson J.M., S. Werres, M. Garbelotto, E.M. Hansen, and D.M. Rizzo. 2003. Sudden oak death and associated diseases caused by Phytophthora ramorum. Online. Plant Health Progress doi:10.1094/PHP-2003-0707-01-DG.
Davidson J.M., A.C. Wickland, H.A. Patterson, K.R. Falk, and D.M. Rizzo. 2005. Transmission of
Phytophthora ramorum in mixed-evergreen forest of California. Phytopathology 95:587-596.
Erwin, D.C., and O.K. Ribeiro. 1996.
Phytophthora Diseases Worldwide. American Phytopathological Society, St. Paul, MN.
Eyre, C.A., M. Konzanitas, and M. Garbelotto. 2013. Population dynamics of aerial and terrestrial populations of
Phytophthora ramorum in California forest under different climatic conditions. Phytopathology 103:1141-1152.
Eyre, C.A., K.J. Hayden, M. Kozanitas, N.J. Grünwald, and M. Garbelotto. 2014. Lineage, temperature, and host species have interacting effects on lesion development in
Phytophthora ramorum. Plant Disease 98:1717-1727.
Fichtner, E.J., S.C. Lynch, and D.M. Rizzo. 2007. Detection, distribution, sporulation, and survival of
Phytophthora ramorum in a California redwood-tanoak forest soil. Phytopathology 97:1366-1375.
Fichtner, E.J., S.C. Lynch, and D.M. Rizzo. 2009. Survival, dispersal, and soil-mediated suppression of Phytophthora ramorum in a California redwood-tanoak forest. Phytopathology 99:608–619.
Frankel, S.J. 2008. Sudden oak death and
Phytophthora ramorum in the USA: a management challenge. Australasian Plant Pathology 37:19-25.
Funahashi, F., and J. L. Parke. 2016. Effects of soil solarization and
Trichoderma asperellum on soilborne inoculum of
Phytophthora ramorum and
Phytophthora pini in container nurseries. Plant Dis. 100:438-443.
Garbelotto, M., and K.J. Hayden. 2012. Sudden oak death: interactions of the exotic oomycete
Phytophthoraramorum with naïve North American hosts. Eukaryotic Cell 11:1313-1323.
Goheen, E.M., E. Hansen, A. Kanaskie, N. Osterbauer, J. Parke, J. Pscheidt, and G. Chastagner. 2006. Sudden Oak Death and
Phytophthora ramorum: a guide for forest managers, Christmas tree growers, and forest-tree nursery operators in Oregon and Washington. Extension Publication EM 8877, Oregon State University. 16 pages.
Goss, E.M., I. Carbone, and N.J. Grünwald. 2009. Ancient isolation and independent evolution of the three clonal lineages of the exotic sudden oak death pathogen
Phytophthora ramorum. Molecular Ecology 18:1161-1174.
Goss, E.M., M. Larsen, G.A. Chastagner, D.R. Givens, and N.J. Grünwald. 2009. Population genetic analysis infers migration pathways of
Phytophthora ramorum in US nurseries. PLoS Pathology 5:e1000583
Grünwald, N.J., M. Garbelotto, E.M. Goss, K. Heungens, and S. Prospero. 2012. Emergence of the sudden oak death pathogen
Phytophthora ramorum. Trends in Microbiology 20:131-138
Grünwald, N.J., E.M. Goss, M.M. Larsen, C.M. Press, and V.T. McDonald. 2008. First report of the European lineage of
Phytophthora ramorum on
Viburnum and
Osmanthus spp. in a California nursery. Plant Disease 92:314.
Grünwald, N.J., E.M. Goss, and C.M. Press. 2008.
Phytophthora ramorum: a pathogen with a remarkably wide host range causing sudden oak death on oaks and ramorum blight on woody ornamentals. Molecular Plant Pathology 9:729-740.
Grünwald, N.J., M.M. Larsen, Z.N. Kamvar, P.W. Reeser, A. Kanaskie, J. Laine, and R. Wiese. 2016. First report of the EU1 clonal lineage of
Phytophthora ramorum on tanoak in an Oregon Forest. Plant Disease 100(5):1024
Hansen, E.M., A. Kanaskie, S. Prospero, M. McWilliams, E.M. Goheen, N. Osterbauer, P. Reeser, and W. Sutton. 2008. Epidemiology of
Phytophthora ramorum in Oregon tanoak forests. Canadian Journal of Forest Research 38:1133-1143.
Hansen, E.M., P.W. Reeser, and W. Sutton. 2012.
Phytophthora beyond agriculture. Annual Review of Phytopathology 50:359-378.
Harris, A.R. and J.F. Webber. 2016. Sporulation potential, symptom expression and detection of
Phytophthora ramorum on larch needles and other foliar hosts. Plant Pathology 65(9):1441-1451.
Hayden, K.J., A. Nettel, R.S. Dodd, and M. Garbelotto. 2011. Will all the trees fall? Variable resistance to an introduced forest disease in a highly susceptible host. Forest Ecology and Management 261:1781-1791.
Kamvar, Z.N., M.M Larsen, A.M. Kanaskie, E.M. Hansen, and N.J. Grünwald. 2015 Spatial and temporal analysis of population of the sudden oak death pathogen in Oregon forests. Phytopathology 105:982-989.
Kline, N., S. Navarro, and D.C. Shaw. 2018. Sudden oak death: prevention, recognition, restoration. Extension Publication EM 9216, Oregon State University. 12 pp.
Magarey, R. D., G.A. Fowler, D.M. Borchert, T.B. Sutton, M. Colunga-Garcia, and J.A. Simpson. 2007. NAPPFAST: an internet system for the weather-based mapping of plant pathogens. Plant Dis. 91:336-345.
Mascheretti, S., P.J.P. Croucher, V.A Vettraino, S. Prospero, and M. Garbelotto. 2008. Reconstruction of the sudden oak death epidemic in California through microsatellite analysis of the pathogen
Phytophthora ramorum. Molecular Ecology 17:2755-2768.
Meentemeyer, R., D. Rizzo, W. Mark, and W. Lotz. 2004. Mapping the risk of establishment and spread of sudden oak death in California. Forest Ecology and Management 200:195-214.
Metz, M.R., J.M. Varner, K.M. Frangioso, R.K. Meentemeyer, and D.M. Rizzo. 2013. Unexpected redwood mortality from synergies between wildfire and an emerging infectious disease. Ecology 94(10):2152-2159.
Parke, J.L. and N. J. Grünwald. 2012. A systems approach for management of pests and pathogens of nursery crops. Plant Dis. 96:1236-1244.
Parke, J.L., and C. Lewis. 2007. Root and stem infection of rhododendron from potting medium infested with
Phytophthora ramorum. Plant Disease 91:1265-1270.
Parke, J.L., E. Oh, S. Voelker, E.M. Hansen, G. Buckles, and B. Lachenbruch. 2007.
Phytophthora ramorum colonizes tanoak xylem and is associated with reduced stem water transport. Phytopathology 97:1558-1567.
Peterson, E., E. Hansen, and J. Hulbert. 2014. Source or sink? The role of soil and water borne inoculum in the dispersal of
Phytophthora ramorum in Oregon tanoak forests. Forest Ecology and Management 322:48-57.
Prospero, S., N.J. Grünwald, L.M. Winton, and E.M. Hansen. 2009. Migration patterns of the emerging plant pathogen
Phytophthora ramorum on the West Coast of the United States of America. Phytopathology 99:739-749.
Rizzo, D.M., and M. Garbelotto. 2003. Sudden oak death: endangering California and Oregon forest ecosystems. Front. Ecol. Environ. 1:197–204.
Rizzo, D.M., M. Garbelotto, and E.M. Hansen. 2005.
Phytophthora ramorum: integrative research and management of an emerging pathogen in California and Oregon forests. Annual Review of Phytopathology 43:309-335.
Rizzo, D.M., M. Garbelotto, J.M. Davidson, G.W. Slaughter, and S.T. Koike. 2002.
Phytophthora ramorum as the cause of extensive mortality of
Quercus spp. and
Lithocarpus densiflorus in California. Plant Disease 86:205-214.
Schweigkofler, W., K. Kosta, V. Huffman, S. Sharma, K. Suslow, and S. Ghosh. 2014. Steaming inactivates
Phytophthora ramorum, causal agent of sudden oak death and ramorum blight, from infested nursery soils in California. Plant Health Progress doi:10.1094/PHP-RS-13-0111.
Swiecki, T., M. Quinn, L. Sims, E. Bernhardt, L. Oliver, T. Popenuck, and M. Garbelotto. 2018.Three new Phytophthora detection methods, including training dogs to sniff out the pathogen, prove reliable. California Agriculture. 72(4): 217-225.
Tjosvold, S.A., K.R. Buermeyer, C. Blomquist, and S. Frankel. 2005. Nursery guide for diseases caused by
Phytophthora ramorum on ornamentals: diagnosis and management. University of California Division of Agriculture and Natural Resources Publication 8156. 20 pages.
Tooley, P.W., and K.L. Kyde, 2007. Susceptibility of some eastern forest species to
Phytophthora ramorum. Plant Disease 91:435-438.
Tonini, F., D. Shoemaker, A. Petrasova, B. Harmon, V. Petras, E.C. Cobb, H. Mitazsova, and R.K. Meentemeyer. 2017. Tangible geospatial modeling for collaborative solutions to invasive species management. Environmental Modelling & Software 92:176-188.
USDA Animal and Plant Health Inspection Service. Phytophthora ramorum and sudden oak death.
http://www.aphis.usda.gov/plant_health/plant_pest_info/pram/index.shtml
Van Poucke K., S. Franceschini, J.F. Webber, A. Vercauteren, J.A. Turner, A.R. McCracken, K. Heungens, and C.M. Brasier. 2012. Discovery of a fourth evolutionary lineage of
Phytophthora ramorum: EU2. Fungal Biology 116(11):1178-1191.
Venette, R.C., and S.D. Cohen. 2006. Potential climatic suitability for establishment of
Phytophthora ramorum within the contiguous United States. Forest Ecology and Management 231:18-26.
Vercauteren, A., M. Riedel, M. Maes, S. Werres, and K. Heungens. 2012. Survival of
Phytophthora ramorum in Rhododendron root balls and in rootless substrates. Plant Pathology 62(1):166-176.
Webber, J.F., M. Mullett, and C.M. Brasier. 2010. Dieback and mortality of plantation Japanese larch (Larix kaempferi) associated with infection by
Phytophthora ramorum. New Disease Reports 22:19
Werres, S., R. Marwitz, W.A. Man in’t Veld, A.W.A.M. de Cock, P.J.M. Bonants, M. de Weerdt, K. Themann, E. Ilieva, and R.P. Baayen. 2001. Phytophthora ramorum sp. nov., a new pathogen on Rhododendron and Viburnum. Mycological Research 105:1155-1165.