Ponomarenko A., S.B. Goodwin, and G.H.J. Kema. 2011. Septoria tritici blotch (STB) of wheat. Plant Health Instructor. DOI:10.1094/PHI-I-2011-0407-01
Septoria tritici blotch (STB)
Mycosphaerella graminicola
Bread wheat (Triticum aestivum L.), durum wheat (T. turgidum [L.] ssp. durum)
Authors
Alisa Ponomarenko1, Stephen B. Goodwin2, and Gert H. J. Kema3
1Department of Botany and Plant Pathology, Purdue University, West Lafayette, IN
2USDA-ARS, Crop Production and Pest Control Research Unit, Purdue University, West Lafayette, IN
3Plant Research International, Wageningen,
The Netherlands
Septoria tritici blotch of wheat
Septoria tritici blotch (STB), caused by the ascomycete fungus Mycosphaerella graminicola (asexual stage: Septoria tritici), is one of the most important foliar diseases of wheat. STB is characterized by necrotic lesions (Figure 1) on leaves and stems that develop after infected cells collapse, and is more prevalent during cool, wet weather. It currently is the most important disease of wheat in Europe and is among the top two or three most economically damaging diseases of this crop in the United States. Extensive applications of fungicides increase the worldwide economic costs attributed to STB.
Symptoms and Signs
The initial symptoms of STB are small chlorotic spots on the leaves that appear soon after seedlings emerge in the fall or spring. As they enlarge, the lesions (Figure 2) become light tan and develop darker colored fruiting bodies (Figure 3). Lesions on mature leaves most often are long, narrow and delimited by leaf veins but also can be shaped irregularly or can be elliptical, particularly on seedlings or leaves that were young when infected. Mature lesions contain black or brown fruiting structures, the asexual pycnidia or sexual pseudothecia. The pycnidia or pseudothecia develop in the substomatal cavities of the host so are spaced regularly within the lesions (Figure 3).
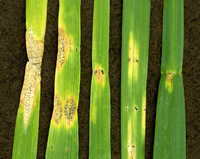 Figure 2 |
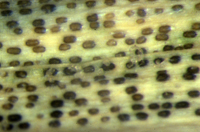 Figure 3 |
STB is found commonly in the same fields and on the same plants as Phaeosphaeria nodorum (asexual stage: Stagonospora nodorum), the causal agent of Stagonospora nodorum blotch of wheat. When both pathogens occur together, they are referred to collectively as the Septoria blotch complex or Septoria complex.
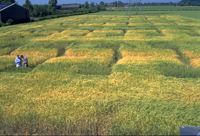 Figure 4 |
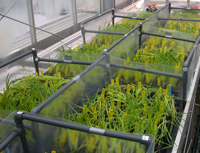 Figure 5 |
Pathogen Biology
Mycosphaerella graminicola is the name of the sexual stage (teleomorph) of the pathogen. The asexual (anamorph) stage, Septoria tritici, was first described as the causal agent of STB in 1842 by Desmazieres. Mycosphaerella graminicola (Fuckel) J. Schröt. was first connected with S. tritici in New Zealand by Sanderson in 1972. This fungus is an ascomycete in the class Dothideomycetes, order Capnodiales, family Mycosphaerellaceae (Figure 6). Mycosphaerella is one of the largest genera of plant-pathogenic fungi and includes the banana black Sigatoka pathogen, M. fijiensis, and many other significant pathogens of important agricultural crops. A close relative of M. graminicola is the speckled leaf blotch pathogen of barley, Septoria passerinii.
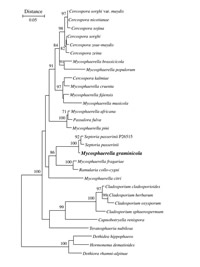
Figure 6
Asexual Reproduction
Asexual spores, or conidia, are hyaline (clear) and threadlike and are produced in specialized structures called pycnidia (Figures 7-9). Each spore typically has 3-7 indistinct septa and measures approximately 2.6 x 62.5 µm. Germination of conidia can be from lateral or intermediary cells. They are exuded from the pycnidia in cirrhi (slimy, tendril-like spore masses), which usually are milky white to buff. There can be many cycles of asexual reproduction during the growing season.
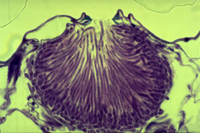 Figure 7 |
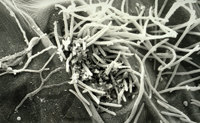 Figure 8 |
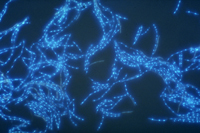 Figure 9 |
Sexual Reproduction
Sexual fruiting bodies, known as pseudothecia, also are produced within lesions. The fungus has a bipolar, heterothallic mating system; individuals of both mating types, designated mat1-1 and mat1-2, must come together to effect sexual reproduction. The pseudothecia are formed underneath the host epidermis. They are globose, dark brown, and approximately 68-114 µm in diameter. Asci, containing ascospores, measure approximately 11-14 x 30-40 µm. The eight ascospores encapsulated by each ascus are hyaline (clear), elliptical, and 2.5-4 x 9-16 µm in size, consisting of two cells of unequal length (Figures 10-11). Ascospores are ejected forcibly from the asci at maturity due to fluctuations of relative humidity following periods of moisture.
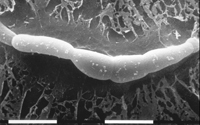 Figure 10 |
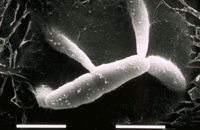 Figure 11 |
Conidia of M. graminicola may germinate in free water from one or both ends or from intermediary cells. Spore germination occurs usually within 12 hours after leaf contact when the humidity is high. At least 20 hours of high relative humidity are needed for successful infection. Wheat resistance or susceptibility does not affect spore germination on the leaf surfaces.
Primary penetration of the leaf is through stomata (Figure 12). Hyphae that enter the stomata are constricted to ~1 µm in diameter but widen after reaching the substomatal cavity. After colonizing the substomatal cavity, hyphae proliferate extracellularly in the mesophyll (Figures 13-15), but do not penetrate these and epidermal cells. Macroscopic disease symptoms generally do not appear before nine days after contact with the pathogen. Mesophyll cells die rapidly beginning around 11-18 days after infection immediately prior to symptom expression.
The lifestyle of M. graminicola is hemibiotrophic. This means it is biotrophic early in the infection process, deriving its nutrition from the apoplast around living cells, then kills the surrounding host cells and becomes necrotrophic (utilizing dead tissue) during the later stages of infection (Figure 16). Infection of wheat by M. graminicola is thus characterized by two stages with at least five phases:
Stage 1 - Biotrophic growth:
i. Initial growth of the hyphae on the leaf surface; 0-24 hours after contact
ii. Host penetration via natural openings, the stomata; 24-48 hours after contact (Figure 12). P>
iii. Intercellular biotrophic phase as hyphae extend within mesophyll tissue and obtain nutrients from the plant apoplast; 2-12 days after contact (Figures 14-15)
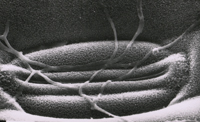 Figure 12 |
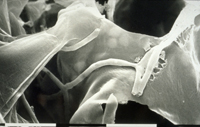 Figure 13 |
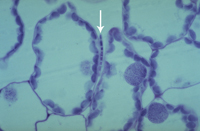 Figure 14 |
Stage 2 - Necrotrophic growth:
iv. A rapid change to necrotrophic growth associated with the appearance of lesions on the leaf surface and collapse of the plant tissue; approximately 12-14 days after contact
v. Further colonization of mesophyll tissue (Figure 16) and formation of pycnidia with conidia in substomatal cavities of senescent tissue; 14-28 days after contact
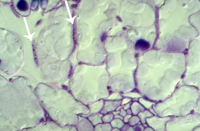 Figure 15 |
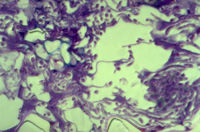 Figure 16 |
The trigger for the switch from biotrophic to necrotrophic growth is not known. During the necrotrophic stage, the hyphae macerate host cells causing collapse. Involvement of a toxin in the switch from biotrophic to necrotrophic growth is suspected but has not yet been proven.
Disease Cycle and Epidemiology
Figure 17
Disease cycle
Infection by M. graminicola is initiated by air-borne ascospores and splash-dispersed conidia produced on residues of the previous season's crop (Figure 17). Primary infection occurs soon after seedlings emerge in fall (for winter wheat) or spring. Ascospore germ tubes are attracted to the stomata, through which they gain entry into the sub-stomatal cavity either directly or after production of an appressorium-like structure (infection cushion). For several days the hyphae grow intercellularly with little increase in biomass.
After the switch from biotrophic to necrotrophic growth, cells collapse, lesions form and are identified initially by small, yellow flecks or blotches. The lesions expand, primarily in the direction of the leaf veins to form long, narrow, necrotic blotches. Pycnidia develop around stomata within the necrotic areas of the lesions and exude conidia in gelatinous, hygroscopic cirrhi. These spores are disseminated by rain splash to leaves of the same or nearby plants. The pathogen survives crop-free periods primarily as pseudothecia but also in pycnidia on crop debris. Autumn-sown crops and volunteer plants can aid survival over winter.
Epidemiology
Initial inoculum usually consists of airborne ascospores, which cause the primary infections on seedling leaves, but also can be from conidia. Primary infections from an ascospore shower will occur evenly over a crop and give rise to lesions that bear pycnidia, the asexual structures that allow for rapid dispersal of the secondary inoculum, conidia.
Secondary spread of STB is by conidia, which form readily in high humidity, particularly if there is free water present on the leaves, but also can be by ascospores. Pycnidia with conidia are produced roughly 14 to 40 days after infection, depending on the host and seasonal conditions. These spores disperse through rain wash and splashing, causing local spread of the disease to uninfected leaves of the same and nearby plants. Production and dispersal of conidia occurs quite rapidly compared to pseudothecia with ascospores, which take several weeks until ripening. Thus both conidia and ascospores contribute to the epidemic but the asexual cycle seems to dominate during the growing season. Ascospores can be airborne over large distances, while conidia are unlikely to travel far from their site of origin by rain-splash dispersal. Conidia help to spread the disease upwards through the canopy. Infection of flag leaves (last leaf to emerge on a wheat stalk) is common and leads to greatly reduced yields and poor quality of harvested grain. Rain splash of conidia can lead to disease foci, which can give a patchy appearance to the overall disease distribution in a field. A more uniform appearance of the disease is typical when the airborne ascospores are plentiful during the initial infection.
Many cycles of sexual and asexual reproduction during the growing season allow epidemics to develop rapidly. Debris from heavily infected leaves and stems remains in fields after harvest to produce inoculum for the next growing season.
Disease Management
Resistant Cultivars
Planting of resistant cultivars is the most economical and simple approach for managing STB. Resistance to M. graminicola can be qualitative (Figure 18) or quantitative and is more common among winter wheat than in spring types. To date, 13 major (qualitative) genes for resistance to STB have been named, mapped and published, and some of them have been found to interact in a gene-for-gene manner with the pathogen. Several others have been identified but not yet published in peer-reviewed journals. In the field, some Stb genes have been quite durable (long lasting) while others have failed due to rapid genetic change in the pathogen population. For example, Stb1 has remained effective in Indiana for more than 25 years, while Stb4 was effective in California for 14 years before it failed, but only lasted one or two years in Oregon. Often wheat cultivars reported as resistant in one region have been found to be susceptible in another. This may be connected to the genetic composition of the local pathogen population, which can be affected by cultivars grown, the suitability of the environment for infection, and the relative importance of the sexual stage in the disease cycle.
Quantitative resistance also is known and may occur commonly in wheat cultivars. However, unlike quantitative resistance against many other pathogens, that against M. graminicola may be isolate specific so could be circumvented more easily. Whenever possible, the use of qualitative Stb genes should be combined with quantitative resistance to help ensure its stability. Molecular markers have been linked to many of the STB genes, which through marker-assisted selection will aid the creation of effective gene combinations in new wheat cultivars.
Figure 18
Cultural Management
Cultural management can reduce the incidence and severity of STB. Rotation to non hosts and sanitation achieved by deep plowing of crop debris can decrease the amount of inoculum available to initiate a new disease cycle. This may be less effective on a field basis, due to long-distance dispersal of ascospores, but may be helpful if coordinated within a region. Late planting of winter wheat (e.g., mid- October versus late September) also may be used as a strategy to moderate the amount of initial infection by avoiding ascospore flights in a newly planted wheat crop.
Chemical Control
Several fungicides are used currently to control STB. A seed dressing of a fungicide, such a triticonazole, can be used in regions where the seedling stage can be affected by the pathogen. Foliar sprays, however, are the most common type of fungicide treatment. Fungicides are only recommended when they would be of economic benefit. Factors to consider include the projected yield and loss from STB and whether the cost of fungicide will justify the expected benefit. The susceptibility of the wheat cultivar and amount of disease, in particular, influence this decision. Monitoring of disease is crucial to assess its progress in the field. Timing sprays to periods when the pathogen is most likely to be active will yield the greatest economic return on effort.
A major problem for chemical control is that many populations of M. graminicola have rapidly evolved resistance to fungicides, especially to the strobilurin class of chemicals. The first resistance of M. graminicola to these compounds was detected in 2002, and populations of the fungus in many regions are now nearly all resistant. Analysis of DNA sequence variation within populations showed that the strobilurin resistance was acquired independently through at least four recurrent mutations of the mitochondrial cytrochrome b gene. Hence, strobilurins are only being used in areas where resistance did not (abundantly) develop yet as these compounds also contribute to a longer green life of flag leaves and therefore to yield. However, the commonest fungicides currently being applied are azoles. The efficacy of these compounds is also decreasing due to mutations in the cyp51 gene, although other factors also may be involved. Alternating fungicides with different modes of action helps mitigate the development of resistance.
Biological Control
Several biological controls are currently being evaluated for STB, and some have shown promise but none is available yet for commercial production. Fungi belonging to Trichoderma spp. have been used previously as biocontrol agents to protect wheat plants against leaf spot diseases in Argentina. A collection of Bacillus megaterium originating from the wheat rhizosphere and leaves, barley, oat chaff, and grain have been screened for their ability to inhibit STB. This bacterium consistently retarded STB development by up to 80% in small-scale field trials. Pseudomonads also have been tested as potential biocontrol agents. Applications of cells of two isolates of fluorescent pseudomonads from soil to wheat seedlings prior to inoculation with M. graminicola markedly reduced symptom expression. In addition, growth of the antagonistic pseudomonads was not affected by a number of commercial fungicides.
Significance
Figure 19
Septoria tritici blotch occurs throughout the world in countries as diverse as Argentina, Ethiopia, Iran, the United States, the Netherlands, Russia, New Zealand, and Australia. It is a big problem on durum wheat in Iran, Tunisia and Morocco. Epidemics can be particularly devastating in developing countries, such as those in East Africa. Severe epidemics of STB can reduce wheat yields by 35 to 50%. In the United States, STB is second only to wheat rust in terms of importance, and it is the number one disease of wheat in Russia and many countries of western Europe. The cost of fungicides to control the disease can be high, and fungicide treatments may not be economical depending on the price of grain. Approximately 70% of the estimated volume of fungicide used on cereals in Europe is used to control STB. In Europe, annual losses from STB are estimated to be $400 million dollars, and similar loss estimates for the United States are more than $275 million dollars per year.
The recent release of the M. graminicola genome sequence is an important step in fully understanding and identifying genes and processes that can be used to help control this important pathogen. The influx of new genomic data will contribute to the elucidation of the genetic and biochemical bases for the host-pathogen interaction. Genomic information can help develop additional molecular diagnostic tools for elucidation of the interactions between M. graminicola and wheat, as well as whole-genome comparisons, genetic mapping, and expression data, which will increasingly help to design improved strategies to manage STB.
Selected References
Agrios, G.N. 2005. Plant Pathology, 5th edition. Academic Press, Inc, San Diego. 922 pp.
Bockus, W.W., R.L. Bowden, R.M. Hunger, W.L. Morrill, T.D. Murray, and R.W. Smiley. 2010. Compendium of Wheat Diseases and Pests: Third Edition. American Phytopathological Society, St. Paul, MN
Goodwin, S.B. 2007. Back to basics and beyond: increasing the level of resistance to Septoria tritici blotch in wheat. Australasian Plant Pathology 36: 532–538.
Goodwin, S.B., C. Waalwijk, and G.H.J. Kema. 2004. Genetics and Genomics of Mycosphaerella graminicola: a model for the Dothideales. Pages 315-330 in: Applied Mycology and Biotechnology. Volume 4, Fungal Genomics. Elsevier Press, San Diego.
Eyal, Z., A.L. Scharen, J.M. Prescott and M. van Ginkel. 1987. The Septoria diseases of wheat: Concepts and methods of disease management. CIMMYT. Mexico, D.F.
Kema, G.H.J., D.Z. Yu, H.J. Rijkenberg, M.W. Shaw and R.P. Baayen. 1996. Histology of the pathogenesis of Mycosphaerella graminicola in wheat. Phytopathology 86: 777-786.
Kema, G.H.J., J.G. Annone, R. Sayoud, C.H. Silfhout, M. van Ginkel, and J. de Bree. 1996. Genetic variation for virulence and resistance in the wheat-Mycosphaerella graminicola pathosystem I. Interactions between pathogen isolates and host cultivars. Phytopathology 86: 200-212.
Kema, G.H.J., R. Sayoud, J.G. Annone, C.H. Silfhout. 1996. Genetic variation for virulence and resistance in the wheat-Mycospharella graminicola pathosystem II. Analysis of interactions between pathogen and host cultivars. Phytopathology 86: 213-224.
Joint Genome Institute, United States Department of Energy and University of California.Mycosphaerella graminicolav2.0 – Home http://genome.jgi-psf.org/Mycgr3/Mycgr3.home.html
Scharen, A.L. 1999. Biology of Septoria/Stagonospora pathogens: an overview. Pages 19-22 in van Ginkel, M., A. McNab, and J. Krupinsky, eds, 1999. Septoria and Stagonospora Diseases of Cereals: A Compilation of Global Research. Proceedings of the Fifth International Septoria Workshop, September 1999, CIMMYT, Mexico.
van Ginkel, M., A. McNab, and J. Krupinsky, eds, 1999. Septoria and Stagonospora Diseases of Cereals: A Compilation of Global Research. Proceedings of the Fifth International Septoria Workshop, September 1999, CIMMYT, Mexico.