Peer-Reviewed by Plant Health Progress
© 2007 Plant Management Network.
Accepted for publication 19 June 2007. Published 6 September 2007.
Tim R. Gottwald, USDA, Agricultural Research Service, US Horticultural Research Lab, Fort Pierce, FL 34945; John V. Graça, Texas A & M University-Kingsville Citrus Center, Weslaco TX 78596; and Renato B. Bassanezi, Fundecitrus, Araraquara, São Paulo, Brazil
Corresponding author: Tim R. Gottwald. tgottwald@ushrl.ars.usda.gov
Gottwald, T. R., da Graça, J. V., and Bassanezi, R. B. 2007. Citrus Huanglongbing: The pathogen and its impact. Online. Plant Health Progress doi:10.1094/PHP-2007-0906-01-RV.
Introduction
Citrus Huanglongbing (HLB), also known as greening disease, has been the subject of several reviews in the past 15 years (14,27,28,33,47), and the reader is referred to them for detailed studies. In this article, we will attempt to present a concise compilation of the main features and symptoms of the disease (Fig. 1), the causal organisms and the vectors including impacts for Florida and Brazil, two relatively new areas where the disease has been discovered, as well as implications for California, Texas, and Arizona citrus industries, which are threatened by the close proximity to the new Florida epidemic, and the rest of the western hemisphere. One aspect that has not been extensively dealt with in previous reviews is the epidemiology of HLB and the implications of temporal increase and spatial spread of the disease on prospects for disease control and mitigation. In addition, considerable new information has been obtained in Brazil relative to damage and potential yield and fruit quality loss that will be addressed here.
|
Fig. 1. An 18-year-old sweet orange tree with an estimated 2 to 3 year defoliation, and dieback. |
|
History, Overview, and Distribution
In the 18th centuryOverview, and Distribution, a severe problem in citrus in the central provinces of India called “dieback” was described (17). In the late 19th century a similar malady was recorded in Assam, India and by 1912 it was a severe problem in Bombay province (17). The cause of this decline was not known. Then in 1919, Reinking (89) briefly described a yellowing and leaf mottle in southern China. It later emerged that farmers in this area of China had been aware of what they called “Huanglongbing” (HLB) since the late 19th century (112). This name has been translated into English as “yellow dragon disease” (47), but Zhao (112) in his 1981 review gives the English name as “yellow shoot disease,” and more recently he (113) confirmed that this is the correct meaning in the Chaoshan district of Guangdong Province where the disease was first observed (66,67). Southern China has long been considered as the area of origin for the Asian form of the disease, but the earlier descriptions of citrus dieback in India suggest that it was present there earlier. Capoor (17) attributed dieback to Citrus tristeza virus (CTV) after biological indexing studies, but Raychaudhuri et al. (86,87) later showed that HLB was a major component of the problem. In 1927, Husain and Nath (49) described a decline and death of citrus in Punjab which they attributed to psyllid feeding damage, but may have been due to HLB, especially considering their description of “insipid fruit” – this may be the first report of an insect being associated with the problem. It now appears likely HLB may have become established in India before being spread to China. Beattie et al. (12) have recently hypothesized that the disease may actually have originated in Africa, possibly in an asymptomatic host such as Verpris lanceolata. It could have been transmitted by an insect to citrus in a European settlement on the east coast of Africa and then taken to the Indian subcontinent in infected plants or budwood 300 to 500 years ago, and then into China later. This might explain why HLB was not reported from China earlier, despite the fact that citrus has been cultivated there for several thousand years (111), and why an HLB-like decline of citrus appeared in Indian writings only in the 18th century (49).
From the 1920s on, new citrus diseases, all with symptoms similar or identical to HLB, were described from several Asian countries. In the Philippines, mottle leaf disease was reported in 1921 (63), “likubin” in Taiwan in about 1930 (85), and phloem degeneration in Indonesia in 1940s (5). HLB had become a serious problem in China by 1935 (66), while in the Philippines, it was not reported as a serious problem until 1957 (75). In Indonesia, 3 million trees were destroyed between 1960 and 1970 (102). In India in the 1960s, HLB was described as causing catastrophic losses (30). In 1929, citrus farmers in South Africa reported a similar disorder (84,106). In the northwest of the country they called it “yellow branch,” while those in the north east, near where a research station had recently been opened in Nelspruit, called it “greening” because of the poor color development of the fruit (106). The name “greening” was adopted by the scientific literature probably because of the proximity of scientists in Nelspruit, and it was only in 1995 when the International Organization of Citrus Virologists held a congress in China that a decision was made to adopt the original Chinese name of “huanglongbing” as the official name (82).
For many years, the disease was considered to be caused by mineral deficiencies/toxicities or water logging, rather than a transmissible pathogen, until Lin (67) demonstrated graft transmissibility in 1956 in China. Later HLB in South Africa was also shown to be transmitted by grafting (78), as well as by the African citrus psyllid, Trioza erytreae Del Guercio (77). Soon thereafter, experiments in India and the Philippines showed that another psyllid species, Diaphorina citri Kuwayama, was the vector of the disease in Asia (18,75).
Prior to 2004, HLB was known to occur in Asia, from Japan in the east, through southern China, Southeast Asia, and the Indian subcontinent to Pakistan. It also exists in the Arabian peninsula, but not in Iran. In Africa, it can be found throughout eastern, central, and southern Africa. The vector, D. citri, has been present in Brazil for over 60 years (48), and has since spread into other South and Central American countries, the Caribbean, and Florida (48,58), and Texas (31) in the United States. Concerns expressed about the threats facing citrus in these and other areas (28) were well founded since HLB has now appeared in Brazil (25,99) and Florida (46). D. citri has also spread recently into Timor-Leste and Papua New Guinea (107).
Symptoms, Types, and Transmission
Symptoms of HLB are varied, and can resemble other disorders. However, there are some unique characteristic features. A tree which becomes infected in the field will usually develop one or more yellow shoots, hence the name of the disease (Fig. 2). If other parts of the tree remain healthy or symptomless, the disease will take on a sectored appearance. The affected leaves develop a pattern of yellow and green areas lacking clear limits between the colors, giving a “blotchy mottle” appearance (79) (Fig. 3). This is the most characteristic foliar symptom and the patterns are asymmetrical on the two halves of the leaf (14) (Fig. 4). Leaves can also become thicker, with veins enlarged and corky in appearance (Fig. 5). In later stages, zinc-like deficiency symptoms can develop (Fig. 6,7), followed by leaf drop and twig dieback. (Fig. 8).
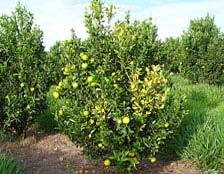 |
|
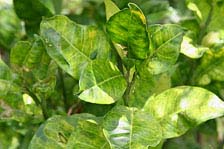 |
Fig. 2. Young sweet orange from commercial planting in São Paulo Brazil with “yellow-shoot” symptom of HLB infection. |
|
Fig. 3. Pummelo foliage from south Florida displaying “blotchy-mottle” symptoms. |
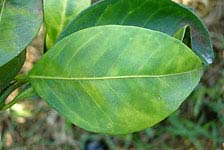 |
|
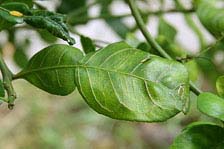 |
Fig. 4. Sweet orange foliage from São Paulo, Brazil demonstrating asymetrical “blotchy mottle” symptom relative to the mid vein. |
|
Fig. 5. Citrus hystrix tree in residential area of Miami showing severe vein corking symptom of HLB. |
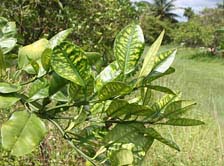 |
|
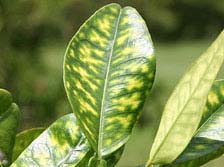 |
Fig. 6. Close up of leaf with zinc pattern deficiency symptom. |
|
Fig. 7. South Florida pummelo tree displaying “zinc-pattern-deficiency” inter-veinal chlorosis symptoms. |
|
Fig. 8. Sweet orange from São Paulo state Brazil displaying disease-induced stem dieback, yellow shoots, and foliar mottling. |
|
There is excessive fruit drop in HLB-infected trees (79) (Fig. 9). Symptomatic fruit are small, lopsided, and as they mature and ripen the stylar end remains green, hence the name “greening” (Figs. 10, 11, and 12). When cut in half, small, dark aborted seed can be observed (Fig. 13), and the vascular bundles in the fruit axis are discolored (Fig. 14). Fruit, especially sweet oranges, can also have a mottled appearance (Fig. 15), and if the peel is depressed with the finger, a silvering of the depressed area will result (Fig. 16)
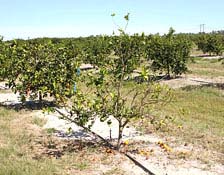 |
|
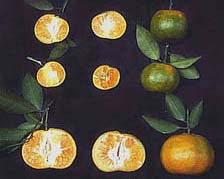 |
Fig. 9. A 2 to 3 year old sweet orange tree in south Florida with HLB-induced fruit drop, dieback, and defoliation leading to thin canopy. Not apparently healthy trees behind. Photo courtesy of Mike Irey. |
|
Fig. 10. Mandarin from Fujian Province, China (PRC). Top two rows: fruits from HLB-infected tree; Bottom row: fruits from healthy tree. Note disease-induced fruit size reduction and mis-shaped (lopsided) fruit. |
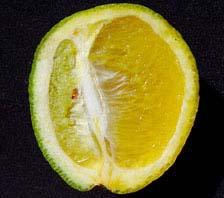 |
|
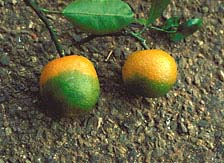 |
Fig. 11. Asymetrical “lopsided” sweet orange fruit from São Paulo, Brazil. |
|
Fig. 12. Mandarin from Fujian Province, China (PRC) displaying stylar end “lack of coloration,” the characteristic leading to the common name for the disease “greening.” |
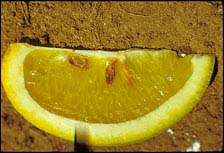 |
|
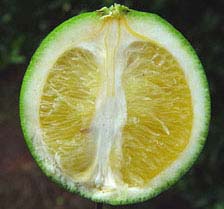 |
Fig. 13. Sweet orange section from HLB infected fruit from China displaying characteristic seed death (brown instead of light beige seed color). |
|
Fig. 14. Sweet orange fruit from Brazil infected with HLB displaying diagnostic orange-brown stain of the vascular columella. |
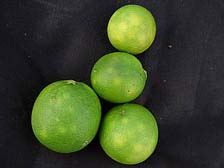 |
|
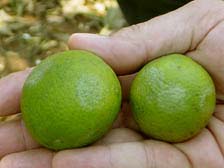 |
Fig. 15. HLB-infected Sweet orange fruit from Brazil with blotchy mottle symptom on fruit surface. |
|
Fig. 16. HLB-infected sweet orange fruit from Brazil with diagnostic “silver spot” that develops when pressed firmly with finger. |
HLB exists as two general types: Asian and African. The symptoms are generally the same, although the Asian form is considered more severe because die-back can be more extensive, and can eventually result in tree death (Fig. 17). The Asian form is also more heat tolerant. Symptoms of African HLB are suppressed by long exposure to temperatures above 30°C. Consequently African HLB is usually found in citrus at elevations above 700 m, while Asian HLB thrives in lower lying, hotter areas. The newly discovered American form in Brazil appears to resemble closely the Asian type of HLB in symptom expression and severity; however, tests in Brazil demonstrate it to be heat intolerant similar to the African type (Silvio A. Lopes, unpublished data).
|
Fig. 17. Orange tree killed by HLB (indicated by red arrow), São Paulo, Brazil. Note other surrounding trees with yellow shoots indicating HLB infection. Photo courtesy of K. L. Manjunath. |
|
HLB in Africa and India is reported to be more severe on mandarins and sweet orange, while lemons, limes, grapefruit, and pummelos are more tolerant (74,83). However, other forms of Asian HLB in Taiwan (97) and that found recently in Florida (98) affect all commercial citrus species severely, as does the new American HLB in São Paulo, Brazil (70). It is possible that strains of the HLB pathogen can adapt to citrus species and cultivars over time and may explain why grapefruit and pummelo were at first considered resistant in areas such as Taiwan but are now considered quite susceptible to HLB including those isolates from Florida.
Some citrus-related plants have been confirmed as hosts for HLB, namely Severinia buxifolia (54), Limonia acidissima (53), and Verpris lanceolata (60). Murraya paniculata has been confirmed as a host for both Ca. L. americanus and Ca. L. asiaticus in Brazil (68,69) (Fig. 18). Beattie et al. (12) have recently commented that there may be some confusion concerning the taxonomic distinction between Murraya paniculata and Murraya exotica, the latter being more susceptible to HLB infection and more attractive to D. citri.
|
Fig. 18. Murraya paniculata tree from Araraquara, São Paulo, Brazil PCR+ for HLB, showing yellowing and thinning canopy. |
|
Natural transmission of Asian and American HLB is by the Asian citrus psyllid, D. citri (18,110) (Fig. 19), while African HLB is transmitted by another psyllid species T. erytreae (77) (Fig. 20). The temperature tolerances and sensitivities of these two species match those of the HLB type they are naturally associated with; i.e., D. citri is heat tolerant, while T. erytreae is sensitive to high temperatures. Under experimental conditions, both species are able to transmit Asian and African HLB (76,62). Adults can acquire and transmit the bacteria, but reports on acquisition times vary widely from 15 to 30 min (18) or 5 h minimum (109) for D. citri to 24 h for T. erytreae (105). Fourth and fifth instar D. citri nymphs can acquire the pathogen which the emerging adult can transmit (109), and there is evidence that T. erytreae nymphs can also acquire the bacteria and that transovarial transmission occurs (105). Once acquired, the psyllid maintains the bacteria and the ability to transmit it throughout its lifespan. HLB can also be transmitted by dodder (Cuscuta spp.) (87). Other species of psyllid have been recorded on citrus, but none have been shown to be vectors (27,47).
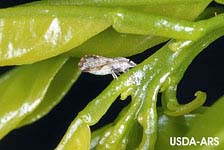 A |
|
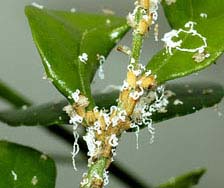 B |
Fig. 19. (A) Adult Asian citrus psyllid, Diaphorina citri, vector of HLB in Asia, and the Americas in typical feeding posture. (B) Adult Asian citrus psyllid nymphs and characteristic waxy feeding exudate. Photos courtesy of David Hall.
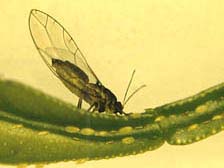 A |
|
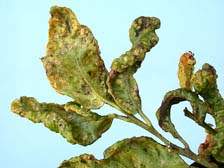 B |
Fig. 20. (A) Adult African citrus psyllid, Tryoza erytreae, vector of HLB on the African continent. Note eggs and nymphs below. (B) African citrus psyllid nymphs and associated feeding foliar deformation. Photos courtesy of S. P. van Vuuren.
Causal Organism, Identification, and Diagnosis
The discovery that HLB was graft- and insect-transmissible led to the conclusion that it was a virus. However, the discovery by electron microscopy (EM) that mycoplasma-like organisms (MLOs) are associated with “yellows” diseases, inspired EM studies on HLB-infected citrus and the report that MLOs were present in the phloem sieve elements of infected plants but not in healthy (61). On close examination, these organisms were seen to have thicker envelopes than MLOs, suggesting that they were true bacteria (32) (Fig. 21). To date, all efforts to isolate the bacterium in pure culture have been unsuccessful (33), but a combination of EM and enzymatic treatments showed the cell wall to be of the Gram negative type (34). Using universal primers, the 16S ribosomal DNA of two strains of the bacterium were obtained by PCR, and comparisons with sequences in GeneBank showed the HLB organism belonged to the α subdivision of the class Protobacteria, but were distinct from other members. The Asian organism has been named Candidatus Liberibacter asiaticus, and the African Ca. L. africanus (35). The new species identified in Brazil has been named Ca. L. americanus (100).
|
Fig. 21. TEM micrograph of Candidatus Liberibacter asiaticus bacteria, causal agent of HLB, within citrus phloem sieve tube. Photo courtesy of Monique Garnier. |
|
Diagnosis based on symptoms can be difficult because of the range of symptoms associated with HLB, although some are quite characteristic, particularly the blotchy mottle leaf symptoms, and the lopsided fruit with green color remaining on the stylar end. Prior to the development of molecular tests, HLB diagnosis was achieved by biological indexing (92), and thin layer chromatography for determining the presence of gentisoyl-β-glucoside (93), although not all HLB-infected citrus species produce it (93,94). The development of monoclonal antibodies held promise for a rapid diagnostic test, but they proved to be too specific for general diagnosis (59). The development of the molecular techniques of DNA hybridization and PCR made it possible to perform rapid, sensitive and specific diagnosis for HLB in the laboratory (101). PCR is now the main confirmatory test and is routinely used in many areas and particularly in Florida and Brazil both for diagnostics and as a prelude to disease management.
Epidemiology
As with many fastidious arboreal plant pathosystems, the epidemiology of HLB has been observed and written about, but only relatively few quantitative epidemiological studies have been conducted. This is due to the perennial nature of the disease requiring a dedication to data collection over multiple years combined with the inability until recently of detection by ELISA or PCR, requiring reliance of past disease monitoring on visual assessment. In addition, there has been difficulty in locating study sites where the disease is allowed to progress unimpeded so epidemics can be followed without intervention of control activities. This is due to the devastating results of infection and the fear of allowing uncontrolled inoculum sources to exist in a region with susceptible plantings (1,2).
From an epidemiological point of view, monitoring the occurrence of symptoms, can be somewhat problematic. The typical leaf mottling and chlorosis similar to zinc deficiency pattern are usually followed by retarded growth, but these symptoms are often non distinct and/or restricted to one branch or side of the tree (5,34,112). Eventually, twig dieback and a general decline ensue due to the pathogens severe effect on the phloem of the host. Fruit symptoms, i.e., small, misshapen, and poorly colored fruit, can also be observed and monitored on diseased trees, especially mandarins and oranges which are considered the most susceptible. Infection by the HLB pathogen is often expressed in sectors on infected trees, indicating incomplete systemic infection or perhaps variable pathogen titer throughout infected trees. The lag in time between transmission of the pathogen by psyllid vectors or by propagation and the onset of visual symptoms can be quite variable depending on the time of the year when infection took place, ensuing environmental conditions, tree age, species/cultivar, and horticultural health, etc. (2,21,39,78,112). Thus visually quantifying the severity or expression of disease symptoms in individual trees is not a true indication of pathogen content. Additionally, due to the temporal variation in symptom expression (symptoms are usually easier to be seen during autumn and winter), trees infected at the same time (especially trees of several years age) may express the onset of infection with great variability over one or more years. This inherently broad and variable lag period, compromises the accuracy of spatial and temporal studies to some extent. Because the visual symptoms we see at any point in time are the expression of infections that have occurred sometime in the past, we are actually visually assessing a “fuzzy history” of infection as it existed at a time period in the past equivalent to the lag period. Nevertheless, with these caveats in mind, useful information can still be gleaned from the epidemiological studies conducted to date concerning the spatial processes that give rise to HLB disease, how it spreads, and how it increases. This information can be used to predict the economic and physical life of a given planting and a means to investigate the influences and efficacy of possible control interventions.
HLB epidemics can be established by introduction of infected plant materials and by transmission due to insect vectors. The unintentional introduction of infected plant materials establishes the disease in new areas or countries and subsequent unregulated movement can have disastrous results. Natural transmission appears to be related to high vector populations and the extensiveness of the inoculum reservoir (1,23). Psyllid migrations appear to be highest when host plants are flushing and psyllid populations sedentary and feeding when foliage is mature (1,19,20). Thus, natural spread is probably greatest in late spring and perhaps other periods when new flush is available and psyllid populations are high (1,20,21). Psyllid vectors are also attracted to yellow wavelengths of light, and thus preferentially to foliage expressing HLB symptoms.
HLB epidemics. The spatial and temporal dynamics of HLB in mature groves have been investigated in only very few cases, primarily from Reunion Island and China (38,39,44) and is only now beginning in Brazil and Florida. In all prior cases, only the Asian HLB pathogen, Ca. L. asiaticus, was known to be present. With the discovery of Ca. L. americanus in São Paulo, Brazil, some data on disease increase and spread of that organism is beginning to be collected as well (10). Prior studies presented an opportunity to investigate the disease in citrus groves in two situations, where inoculum was introduced by infected planting stock, and where clean stock was utilized and the pathogen was introduced by the immigration of bacteria-contaminated psyllid vectors. The studies were conducted to establish preliminary rates of disease increase of citrus HLB under endemic conditions in the presence of vector populations, and develop preliminary temporal models to estimate the expected longevity of infected sweet orange and mandarin groves.
To accomplish this, citrus HLB disease was monitored by visual assessment in citrus plots over a period of several years in four locations (10). The Reunion Island plot was located on the coastal leeward side of the island. The plot was established from disease-free “Valencia” sweet orange plants in 1970 originating from a French budwood program in Corsica. The Liuzhou Citrus Farm Plot (LCF) was located near Liuzhou City, Guangxi Province, People’s Republic of China. This plot was established in October 1953 and consisted of a mix of “Xinhuicheng” and “Anliucheng” sweet orange, on Rangpur lime. The Liuzhou Agricultural Research Institute plot (LARI) was established in Spring 1968 and was also located near Liuzhou City but was originally designed as a rootstock trial, and consisted of “Xinhuicheng” and “Anliucheng” sweet orange, “Tankan” mandarin, and “Ponkan” tangerine on various rootstocks. The two Liuzhou, China, plantings were probably established from a very low incidence of HLB-infected planting material although no greening symptoms were noted at planting.
Minimal insect control programs were practiced in the plots in China and, therefore, they were probably not sufficient to adequately control or affect the vector populations to an appreciable extent (39). The same was true for the Reunion Island plot where, although an insecticide program was practiced, psyllids were not a primary target insect (8). The Asian psyllid, D. citri, and the African psyllid, T. erytreae, were present on Reunion Island during the duration of the experiment. However, D. citri was the only psyllid present in the lower, hotter coastal area where the Reunion Island plot was located (1,2) (Fig. 22), and only D. citri, is presently known to exist in the PRC where LCF and LARI plots were located (112). Prevailing wind direction in the two China plots was variable, however, prevailing winds in the Reunion Island plot were east to west. Disease was also assessed in an additional plot in the Poutai Citrus Cooperative Grove near Shantou, Guangdong Province, PRC in 1986 and again 1988 for spatial analysis of vector spread only (38). The Shantou cooperative plot consisted of ca. 20,000 trees of Tankan/Fuzhou mandarin on land converted to citrus from swamp rice and received only a minimal range of care with primitive, hand-fashioned, bamboo, hand sprayers for insecticide treatment. The trees were planted on beds running north-south and the planting was established from disease-free planting materials in 1983. Although the data from these plots was published previously (38), new analyses are presented here as well which provides greater insight into increase and spread of HLB.
|
Fig. 22. Graphic diagram of Reunion Island, a volcanic island in the Indian Ocean. Diagram demonstrates distribution of two psyllid vectors of HLB relative to elevation and temperature. |
|
Disease severity and disease incidence were visually assessed for each tree in Reunion Island during 1975, 1977, and 1979 (2,6,8). To estimate disease severity, each tree was divided into an upper and lower hemisphere and each hemisphere was subdivided into four equal quadrants resulting in eight sections per tree. Sections were scored individually on a 0 to 5 scale that was indicative of the proportion of limbs expressing disease symptoms and resulted in a severity rating of 0 to 40 for each tree on each survey date (2,39) (Fig. 23). Disease incidence at the tree level was calculated as the proportion of trees expressing HLB symptoms. HLB confirmation was periodically done via (i) indexing by side grafting suspect shoots onto seedling mandarin indicator trees in the greenhouse and incubating these grafted trees for several months, and (ii) by examining prepared foliar specimens via transmission electron microscopy for bacteria-like organisms in the phloem sieve tubes (2,39). For the LCF, LARI, and Shantou plots, only disease incidence was assessed by careful visual examination of all trees within the plots for HLB symptoms (38,39).
|
Fig. 23. Diagram of a citrus tree divided into 8 sections and used for HLB disease severity estimation or 0 to 5, within each section. Method used for visual assay of individual trees on Reunion Island HLB epidemiology plot. |
|
Analysis of disease progression. As explained above, HLB epidemics are multiyear in duration and it would be a rare instance that a planting would be allowed to progress to a high disease incidence before it would become nonproductive and be removed. Therefore, most of the HLB temporal data sets do not include assessments near a disease asymptote (39). Because of the lack of asymptotic data, either the exponential or the logistic models adequately described disease progress in these plots over time (Fig. 24). In one case an asymptote of disease was reached in the LCF plot after 13 years. Because the groves became unproductive due to severe HLB infection and the trees were removed, disease incidence only reached 0.76 after 6 years and 0.84 after 9 years for LARI and Reunion Island, respectively. Even so, the logistic model was used to predict asymptotic levels of disease (0.98 to 0.99) after ca. 6 to 7 and 12 to 13 years after planting for LARI and Reunion Island plots, respectively (39). Logistic rates of disease increase were higher for LCF and LARI when compared to the Reunion Island plot (39). This may be because vector populations were much greater in the Liuzhou City, China area where the disease and vectors have been endemic for many years compared to Reunion where citrus is not as widely planted and the disease and vector were more recent immigrants. These rates of HLB disease increase are comparable to those for other graft-transmissible disease of citrus such as the CTV/Aphis gossypii pathosystem, and the citrus variegated chlorosis (CVC)/sharpshooter pathosystem (41,42,43,45). For the Shantou plot, a small planting of 24 citrus trees associated with a small vegetable garden was located immediately adjacent to a farmhouse on the southern edge of the planting. These trees were all severely infected with greening and did not originate from disease-free nursery stock as did the rest of the planting. HLB infection in the planting was very rapid and increased from only 16 trees (0.08%) in 1986 to 2880 trees (14.4%) in 1988 (Fig. 25). Unfortunately, HLB progress was not followed beyond 1988 (38).
|
Fig. 24. Analysis of disease progress of citrus huanglongbing in citrus groves in the People's Republic of China and Reunion Island. LCF Liuzhou Citrus Farm Plot located near Liuzhou City, Guangxi Province, People’s Republic of China. LARI = Luizhou Agricultural Research Institute, Guangxi Province, the People's Republic of China; RI = Reunion Island; R2 coefficient of determination; b = slope of regression. Disease percentages were transformed by ln (y) and ln (y/(1-y)) for exponential (EXP) and logistic (LOG) models, respectively. |
|
|
Fig. 25. Plot map of the Shantou HLB epidemiology plot in China (PRC). Vegetable garden was located to south with 6 to 7 HLB-infected trees that existed prior to establishment of the commercial citrus planting. White dots indicate position of mandarin citrus trees. Red dots indicate initial visual assessment of spatial distribution of HLB (16 infected trees) in 1986. Larger white dots indicate distribution of HLB in 1988, 7,425 HLB-infected trees, small white dots are visually non symptomatic trees. |
|
In other studies carried out in South Africa (African HLB), Vietnam (Asian HLB), and Brazil (American HLB), in areas where the disease is endemic and have large numbers of inoculum sources, even with insecticide sprays, faster HLB epidemics in younger groves were observed. According to Catling & Atkinson (22), 50 Valencia trees on rough lemon rootstocks originating from a nursery in the Swaziland lowveld, where HLB symptoms were unknown, were planted at Malkerns Research Station (Swaziland) in December 1967. Vector populations of the African citrus psyllid, T. erytrae, were controlled by insecticide sprays when it reached 0.5 colonies per tree during the first year after planting, but higher populations were allowed thereafter. Foliar symptoms first showed at 7 months. HLB-symptoms incidence was 0.24 (= 24% of trees diseased) at 11 months, 0.42 at 20 months, and 1.00 at 43 months (22). In South Vietnam, the high pressure of inoculum and psyllid vector presented were the cause of a very fast epidemic in orchards established in 2003 (37). Three years after planting, incidence of HLB reached 0.96 in the orchard managed without any insecticide. Even in the orchards with conventional insecticide practice (fortnightly spraying of Fenobucarb) and systemic insecticide (monthly trunk applications of Imidacloprid), the incidence of HLB reached 0.74 and 0.24, respectively three years after plantation (37). In São Paulo Brazil, the incidence of HLB-symptomatic trees in four citrus blocks planted in 2002, surrounded by older heavily HLB and CVC affected blocks, varied from 0.004 to 0.20 in 2004. After one year, the disease incidence in those blocks reached 0.26 to 0.40, however there was no CVC symptomatic trees (R. Bassanezi, unpublished data). At the same farm, other citrus blocks planted in 2000 showed a disease incidence of 0.24 in 2004 and 0.70 in 2005 (R. Bassanezi, unpublished data). A similar block is shown in which HLB disease incidence increased from 0.06 to 0.274 in 9 to 10 months (Fig. 26). In a large commercial planting in South Florida, HLB increased from 0.002 to as much as 0.39 disease incidence in 10 months (M. Irey, unpublished data).
|
Fig. 26. Hamlin sweet orange planting in São Paulo state, Brazil demonstrating rapid spread of HLB over 9 to 10-month period. White squares are healthy trees, red squares are HLB-diseased trees determined via visual assessment. Older commercial citrus blocks to the north and west were heavily infected with HLB which acted as inoculum sources for new infections within the block. |
|
Spatial analysis of HLB data. Spatial pattern analysis is used to better understand the relationships among infected trees near and at distance to each other and thereby gain some understanding of vector spread of HLB. This is accomplished by examining the HLB data at various spatial levels (hierarchies) including, (i) immediately adjacent trees within and across row, (ii) within groups of trees, and (iii) among groups of trees over some distance to each other (50,52). Although insufficient time has elapsed since the discovery of HLB in Brazil and Florida to determine the temporal increase of the disease, many maps of the disease have been compiled from visual surveys, especially in Brazil where over 155 maps now exist. These have been analyzed at various spatial scales to determine the spatial pattern of the disease as it occurs in Brazil and attempt to draw some conclusions concerning spread (10). The results of this work in the Western hemisphere mirror that previously found in Reunion and Asia.
Some evidence of aggregation (clustering) among immediately adjacent diseased trees was demonstrated by the ordinary runs analysis, which is a unidirectional analysis, in all plots tested, but this was not particularly strong (10,38,39,40,73) (Table 1). In many cases there was some orientation or direction to aggregation in most plots indicating that within-row aggregation (where trees are planted closer together) was slightly stronger than across-row. This directional orientation for aggregation was best demonstrated in the Shantou plot, where a higher degree of aggregation was indicated (155/199 = 77.9% of rows tested) in the north-south direction compared with (91/205 = 44.4% of rows tested) the east-west direction (Table 1). This greater north-south aggregation was in the same orientation as the raised planting beds. For the Reunion Island and Shantou plots, mapping of isopaths of disease severity demonstrated higher concentrations of disease initially around the perimeter of the western half of the Reunion Island plot and the southern edge of the Shantou plot (Figs. 27 and 28). For the large number of maps of HLB incidence from Brazil, aggregation among HLB-symptomatic trees was detected by ordinary runs analysis, and clustering existed in both within- and across-rows directions. However, as with the Reunion and China data, the percentage of aggregation within- and across-rows was low (10).
|
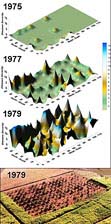 |
|
Fig. 27. Response surface representations of disease development and spatial spread of citrus huanglongbing disease in Reunion Island. The citrus grove was established in 1970. Assessments of disease severity were conducted in 1975 (top), 1977 (middle), and 1979 (bottom) panels, respectively. Note high disease severity around perimeter of western half of grove in 1975, early in the epidemic followed by spread and increase to the east. Final panel is an aerial photo of the Reunion Island HLB plot. Photo is in a similar orientation as the response surface maps in Fig. 2 and was take about the same time as the final disease assessment in 1979. (Photo courtesy of Bernard Aubert). |
|
|
Fig. 28. Response surface representations of disease development and spatial spread of citrus huanglongbing disease in the Shantou Citrus Cooperative plantation near Shantou, Guangdong Province, PRC in 1986 (top) and again 1988 (bottom). Note high disease incidence was predominantly along the southern border of the planting in 1986 and apparently emanated from dooryard plantings immediately to the south. Subsequent spread was to the north in the direction of equipment and personnel travel along north-south oriented raised beds. |
|
The association of diseased trees within groups was examined by Beta Binominal Analysis (BBD) as an overall assessment of heterogeneity of disease incidence. When fitted to disease data parsed into quadrats, the superior fit of the beta binomial distribution is indicative of an aggregated pattern (51,72). Aggregation at the group spatial level was demonstrated for all plots at all locations and all quadrat (group) sizes for the majority of years. The only exceptions occurred when disease incidence was extremely low or high as expected (38,39). In Brazil, the binomial index of dispersion for various quadrat sizes suggested aggregation of HLB-symptomatic trees for about 40% of the plots tested (10).
Spatial autocorrelation was used to examine the relationship among groups of trees over longer distances (45,73,80). The LARI, LCF, Shantou, and Brazil plots were examined at various group sizes. For the LARI plots, groups of trees were associated with one another up to four groups away within and across rows indicating associations as large as eight trees within and across rows. Discontinuous (reflected) clusters were also seen at oblique angles as far as 14 to 16 trees apart. The LCF results were somewhat different. Only immediately adjacent groups were significantly associated with one another. However, numerous reflected clusters also existed, some at oblique angles and as far as 18 tree spaces apart. In São Paulo, Brazil, data from plots ranging in disease incidence indicated in 14 of 20 cases, clusters of HLB-infected trees were found to be associated with secondary clusters whose centers were at distances ranging from 4.2 to 22.1 tree spaces distant. Thus for all of these locations, there are indications of psyllid vector movement resulting in transmission both to nearby trees causing clusters and to trees at considerable distance initiating new foci of infection. The Shantou plot was more extensive in size and allowed HLB data to be examined on a larger scale. Significant aggregation was seen at all quadrat sizes examined and core clusters were very large, demonstrating continuous relationships among groups circumscribing areas of local influence of 300 to 1,672 tree spaces. Numerous reflected clusters existed as well, from 8 to 572 trees in size located 25 to 30 m from the core cluster and demonstrated spatial relationships among many clusters of diseased trees within the planting (Fig. 26). The Reunion Island plot was much smaller in size and groups of adjacent trees were associated with each other up to one group away across rows and two groups within rows but discontinuous groups were also found up to seven groups (14 trees) away. This demonstrates the development of areas of local influence up to 8 tree spaces (~24 m) in radius where incomplete aggregations of trees existed. However, discontinuous groups of trees also were found to be associated with each other as far as 8 to 16 tree spaces (~24 to 48 m) apart (Fig. 27).
Spatial and temporal conclusions and interpretations. The results of spatial analysis of the large Shantou grove were very enlightening (38). A high proportion of aggregation was detected in the planting by ordinary runs analysis and was much higher 77.9% in the north-south orientation along rows compared to 44.4% in the east west orientation across rows. This was probably due to the topographic conditions within the planting. Because of the high and narrow beds on which the grove was planted and the deep irrigation ditches between rows running north-south, traffic was entirely restricted to this direction. Psyllids, when disturbed, often move to the next tree or even many trees away in the opposite direction from the disturbance. Infective vectors entering the grove from the south would have been disturbed and moved along the rows primarily to the north. This would account for the predominance in clustering within north-south oriented rows (Figs. 25 and 28). The secondary clumps or clusters of diseased trees determined by the spatial autocorrelation analysis were also oriented in this direction from one another. Vector movement appears to be generally from one tree to the next; however, there is some indication that longer distances are traversed at times and new foci of disease initiated. This could possibly be stimulated by psyllid population crowding, or major disturbances due to horticultural practices, combined with insufficient insecticide control. The distance between groups of disease trees was estimated to be 25 to 30 m, indicating psyllids often flew at least this distance when disturbed or stimulated to fly.
Disease-free planting material was utilized to establish the Reunion Island plot. However, greening-infected groves and D. citri were prevalent in the vicinity of the Reunion Island during the course of this experiment (2,39). It is likely that migrating, infective D. citri populations carried the greening pathogen from nearby infected plantings to the Reunion Island planting early in the life of the planting. However, sufficient symptoms for recognition of the disease did not develop until 5 years after the plot was established, when visual estimation determined 9.5% of the trees were already infected with HLB. Due to the delay between vector transmission and symptom expression, it is likely that the bacterium had already infected numerous other trees but the disease was visually subclinical in those trees at that time. Because HLB incidence and severity were greater around the perimeter of the western half of Reunion Island, the bacterial pathogen may have been introduced by pathogen-carrying psyllids from infected groves to the west in spite of east to west prevailing winds (39). Early in the epidemic, a high degree of aggregation of diseased trees was detected by ordinary runs analysis and spatial autocorrelation in the east-west axis of the plot (Tables 1 and 2), and by surface response map (Fig. 27) indicating disease movement in that orientation. Vector migration would have been against the prevailing wind and, therefore, may have represented active migration of the vectors rather than passive transport due to wind.
|
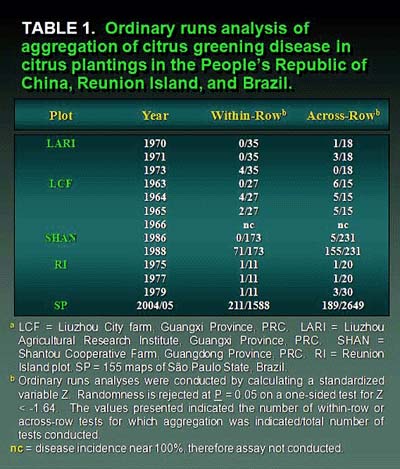 |
|
|
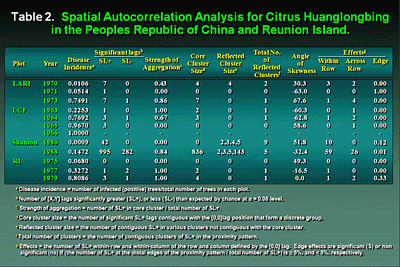 |
|
The introduction of diseased planting materials into LARI and LCF plots was likely to have been fairly random, although disease aggregation was detected by ordinary runs, except at very high and very low disease proportion (Table 1). Spatial autocorrelations also demonstrated decreasing cluster size over time (Table 2).
From a temporal point of view, the relative increase of the HLB disease is slow and multi years in duration, even when vector populations are high and inoculum sources are prevalent. One interpretation is that vector transmission in the field must therefore be inefficient relative to other vectored plant diseases such as vegetable and field crop virus diseases that can reach asymptotic levels within a single season. Relatively slow epidemics are common among vectored diseases of citrus as well as other arboreal pathosystems even though vector populations for some diseases such as HLB and CTV are often very high.
From a spatial perspective, results of the various spatial analyses conducted to date can be interpreted in combination to obtain a more comprehensive picture of spatial patterns that existed in HLB-infected plantings. The combined analyses indicate two mechanisms of vector spread of HLB, within local areas and over longer distances. Within local areas, aggregations of infected trees occur that at times can be quite large, i.e., encompassing as many as 1672 trees. This does not mean that every tree in these local areas will become infected, but that a high proportion of them will be as demonstrated by the strength-of-aggregation calculation associated with spatial autocorrelation results and define a focus of infection (Table 2). In this case, vectors are apparently spreading the pathogen to either adjacent or nearby trees only a few spaces away. Spatial autocorrelation also identifies a prevalence of reflected clusters or areas of aggregation that are discontinuous with the main cluster. These are interpreted as indicative of the presence of secondary foci. These are quite prevalent and are at a distance of about 25 to 50 m from the main cluster of disease and each other. Such a pattern of widely spaced foci perhaps indicates a spatial mechanism associated with longer distance vector movement. That is, when vectors move, either naturally in search of new feeding opportunities or when disturbed as in the Shantou plot, they occasionally do so to other than nearby trees and when this occurs they move at least 25 to 50 m. Longer or regional scale vector transmission has not been investigated. It is obvious that this likely happens as well, but is beyond the scope of the studies conducted to date.
Enhancement of analysis of distribution and spread via new detection methods. The spatial and temporal data presented were collected by visual assessments, and thus a history of infection as it existed at some point in the past. It is probable that new detection methods will improve our assessment of disease incidence data, and thus our understanding of disease increase and spread significantly. Serological methods and PCR are to some extent reliant upon pathogen titer in a plant and its distribution relative to where samples are taken (36). In many cases, serological detection is nearly coincident with visual symptom manifestation. The non-uniform distribution of pre- and even post-systemic infections of the HLB bacteria, means that even if a tree is infected, samples collected from a portion of the tree with low or no titer, will yield a negative assessment. At present PCR is both complex and time consuming. At this point in time the ability to process the thousands of samples necessary to track an epidemic remains manpower and cost prohibitive but significant advances may make such endeavors possible in the future. However, in a recent experiment in south Florida, RT-PCR was used to map HLB infections in six 14 × 14 tree plots to date (55). The results are quite elucidating relative to asymptomatic infections. The authors found nearly the same number of asymptomatic but RT-PCR positive trees within the plots as visually symptomatic trees (55). To take this thought process a step further; intuitively, we know that it requires some time after vector transmission of the bacteria for the bacterial populations to build up locally and become distributed within an infected tree. Thus there is an additional latency period between infection and sufficient bacterial population increase and distribution before it is likely to detect asymptomatic HLB infections via PCR. It also requires some serendipity to inadvertently collect asymptomatic samples for PCR assay from a portion of the tree with bacterial infection. Thus, although PCR allows us to detect many asymptomatic infections, we are still only detecting a portion of the more recent but asymptomatic infections in the planting. There is likely some unknown number of infections still beyond our ability to detect. At present we do not have the ability to detect an infection soon after vector transmission. So we know that for any visual assessment of disease incidence there is at least double that number of infections plus an additional population of infected trees that remain subclinical relative to PCR assay. This information is quite useful and may become a clue for future disease management and decision making strategies relative to the productivity of a planting. That is, knowledge of the visual disease incidence provides a basis on which to estimate the actual disease incidence in the block. It also provides a means to determine a threshold of disease incidence beyond which it would be more economically beneficial to remove an infected block and replant with disease-free trees than to continue to attempt to manage the disease in that planting, knowing that there is considerably more disease than meets the eye.
A second finding of the study was the distribution of asymptomatic but RT-PCR positive trees relative to visually symptomatic trees (55) (Fig. 29). Although the plot sizes were small, by applying a nearest-neighbor analysis it was determined that about 90% of the visually negative but RT-PCR positive trees occurred within approximately 38 m (125 ft) of a visually positive tree (Fig. 30). This indicates that there is a considerable amount of secondary spread that although not necessarily nearest neighbor, still occurs predominately within a relatively short distance or “area of influence.” This distance also corroborates the findings of spatial autocorrelation that indicate the establishment of secondary foci within 24 to 50 m as described above.
|
Fig. 29. Spatial Patterns of visual and PCR analyses in five HLB-infected plots in south Florida. Medium blue indicates plants with visual symptoms of HLB and RT-PCR positive, dark blue indicates plants with visual symptoms but RT-PCR negative, and light purple indicates plants without visual symptoms and RT-PCR positive. |
|
|
Fig. 30. Cumulative assessment of spatial patterns of visual and PCR analyses in five plots in south Florida. (Top) Nearest-neighbor distance between visually HLB symptomatic trees and HLB trees that are RT-PCR positive parsed into 25 ft categories. (Bottom) Cumulative distribution of nearest neighbor distance indicated (at top) with indication that 90% of RT-PCR positives occurred within 125 ft of a tree with visual symptoms. |
|
Known Regional Distribution of HLB in the Western Hemisphere
In Brazil, both Asian and the newly described American HLB were first discovered in 2004 near the city of Araraquara in the state of São Paulo, near the center of commercial citrus production in that state which is the largest citrus producing state in Brazil. Subsequent surveys have indicated that the highest incidence of disease is also centered in this area providing indirect evidence that this area is the most probable point of introduction (Fig. 31). An overall assessment of the aspect of the disease in the area and of trees believed to have the oldest infections, lead researchers there to believe that HLB was likely introduced into the area approximately 10 years ago. Simple measurement of the distance from the presumed point of introduction to the advancing edge of the epidemic results in an estimated distance of spread of approximately 120 mi, or about 12 mi per year. As of March 2007, HLB-symptomatic and PCR positive trees were found in 122 municipalities of São Paulo State and one of Minas Gerais State. We must also consider that the disease has undoubted spread beyond the area of visual detection by some distance as well. However, this does not take into account that the disease may have been transported inadvertently and established in new locations by contaminated nursery or planting materials prior to realizing the disease existed in the area. Production of nursery trees under insect proof condition in State of São Paulo has begun in 1999, but was only mandatory after January 2003. This would mean that human assisted long distance transport was playing a role and thereby would decrease the estimated yearly distance of spread by some unknown amount. Never the less, this is indirect evidence of long distance transport of HLB by contaminated vectors as well. Up to May 2006, 5,000 samples were collected and sent by growers to Fundecitrus, Araquara, Sáo Paulo, Brazil. Ca. L. americanus is the prevalent species in São Paulo state, comprising 77.2% of all positive PCR samples, against 21.4% for Ca. L. asiaticus. The remaining 1.4% samples were positive for both species (108). American HLB is also more widely disseminated than Asian HLB in Brazil. At present, 65% of HLB-affected municipalities have only Ca. L. americanus, 33% have both Ca. L. americanus and Ca. L. asiaticus, and 2% only Ca. L. asiaticus alone (108) (Fig. 32). That scenario could be explained by two hypotheses: (i) American HLB originated in Brazil, and so is more adapted than Asian HLB; (ii) Asian HLB was introduced in Brazil after American HLB; (iii) Asian and American HLB were simultaneously introduced but either the American HLB isolate has a competitive advantage in the Brazilian citrus ecosystem, or American HLB was inadvertently propagated preferentially, or American HLB is more efficiently transmitted by psyllids.
|
Fig. 31. Distribution and incidence of HLB in 123 municipalities of São Paulo State, Brazil as of March 2007. The incidence was calculated base on data from Official HLB Eradication Campaign (Fundecitrus)./P> |
|
|
Fig. 32. Distribution of Candidatus Liberibacter americanus and Ca. L. asiaticus in São Paulo State, Brazil as of March 2007. The occurence of each bacterial species was based on samples sent to Fundecitrus by citrus growers. |
|
In Florida HLB was first discovered by a Florida Department of Agriculture and Consumer Services scientist on 24 August 2005 during a routine CAPS survey in a pummelo tree in a commercial tropical fruit nursery in Florida City and immediately thereafter in the residential communities of Pinecrest and Coral City (Fig. 33). Presumptive PCR positive samples were sent to the USDA, APHIS diagnostic lab in Beltsville and confirmed on 2 September 2005 to be Candidatus Liberibacter asiaticus the causal agent of Asian HLB. The discovery immediately prompted a survey to delimit the infection. East-west transects were surveyed every 5 mi northward in an attempt to determine the northern extent of the disease distribution (Fig. 34). A positive detection in a transect immediately prompted a survey in the next transect 5 mi to the north. HLB distribution was quickly confirmed to extend northward to the Fort Pierce residential area, 120 mi. Concern for the commercial citrus industry prompted simultaneous selected survey of commercial plantings nearest to the known residential distribution along the southeast Florida coast. Suspect samples from selected commercial plantings also quickly revealed the presence of HLB. This prompted a state-wide spot survey of the entire Florida commercial citrus industry and selected residential areas. At the time of this writing, the known distribution has been determined includes 23 counties, predominately in the southern half of the Florida peninsula (Fig. 35). Preliminary indications are that the disease is most severe in the southern most residential and commercial areas. This would imply that the initial introduction(s) may have been in this area. However, unlike Brazil, there is no clear indication of a point or even a limited area of introduction, although it is presumed to have been introduced somewhere in the southeastern metropolitan residential area. Based on this assumption, measurements from the initial discovery point and the most nearest residential infections to the most adjacent commercial planting are approximately 90 and 55 mi, respectively (Fig. 34). This nearest commercial planting is both isolated and has not acquired nursery materials from outside sources. Thus the assumption is that HLB arrived in this planting via psyllid vector dissemination and transmission. This is further suspected because the distribution of HLB symptomatic trees is more intense in the southeastern portion of this planting proximal to the residential area and separated from it by a citrus-free void of march, i.e., the Everglades area. Although not conclusive, it is further possible evidence that long distance movement of HLB is likely by psyllid vectors and in this case could be related to movement of air masses carrying infected vectors during hurricane or tropical storms that have affected Florida recently over this void to commercial areas.
|
Fig. 33. Points of initial discoveries of HLB in South Florida. First discovery was an infected pummelo tree adjacent to a mixed planing of tropical fruit trees in Florida City area immediately followed by a discovery of an infected Citrus hystrix at residential property in Pinecrest area of Miami. |
|
|
Fig. 34. Initial HLB survey map demonstrating 5-mi incremental survey as it progressed northward up the south east coast of Florida from the initial Florida City and Pinecrest discoveries, in an attempt to delimit the disease incursion. It was immediately apparent that the disease has already spread and become established alone the residential southeast coast of Florida as far north as Stuart. The survey then turned to the nearest commercial plantings, located in southeast Hendry Co., separated from the residential area by the Florida Everglades swamp (a void with no citrus), and HLB was confirmed in this area as well. Measurements indicate the approximate distance between these commercial infections and the initial discovery in Florida City (90 mi) and to the nearest residential discovery west of Davie (55 mi). |
|
|
Fig. 35. Current confirmed distribution of HLB in commercial citrus and residential properties in the State of Florida as of 19 June 2007. PCR confirmations of the disease have identified HLB in 15 counties in southern Florida. However, the map does not represent the much greater number of HLB visual thousands of additional detections that are not PCR confirmed. Map courtesy of USDA, Animal and Plant Health Inspection Service and Florida Department of Agriculture and Consumer Services. |
|
The formation of an HLB science panel composed of scientists and regulatory officials with knowledge of the disease has recommended a more thorough and systematic survey of the entire Florida commercial industry to both delimit the extents of HLB within the industry and estimate the incidence of infection. In response, the USDA, ARS has developed a stratified-weighted survey to be deployed based on the known GIS distribution of commercial plantings and there estimated susceptibility based on cultivar content. The survey stochastically selects an adjustable proportion of plantings to be sampled within each 36-mi² area (township) depending upon the manpower and resources that can be dedicated to the survey and the duration of time during which the survey is desired to be completed. At present the USDA APHIS has deployed the survey in three successive sweeps of the Commercial industry, each requiring three months to complete a ~15% sampling of all commercial blocks. The survey is transportable to other commercial citrus industries and will be provided to California, Texas, and other citrus producing states to survey their citrus plantings as well. The survey has also been adapted to include multiple diseases (HLB, citrus canker, and citrus variegated chlorosis) in an attempt to use a single “multi-pest” survey protocol to detect more than one exotic pathogen concurrently.
HLB Damage: Yield and Quality Loss Estimates
There is no exaggeration when HLB is described as the most important, severe, serious, destructive, devastating disease of citrus in the world (14). The source of resistance for HLB is unknown. Management of HLB is very hard and expansive. Where the disease is endemic or there is no effective control by reduction of bacteria inoculum and psyllids, the disease progression in the orchard could be relatively fast, reaching more than 0.95 of incidence in 3 to 13 years after the first symptom onset (8,15,22,37,38,39). Once infected, there is no cure for the diseased tree, even after a drastic pruning at trunk level (71), and the evolution of symptoms severity can be very quick until the symptoms are distributed throughout the tree canopy and become very severe. Severe symptoms have been observed, both in Africa and Asia, 5 to 8 years after planting (4,6,8,13,88,90) or 1 to 5 years after onset of the first symptoms, depending of tree age, variety, and orchard management (5,66,95). The damage due to HLB is considerable. The diseased tree declines, yield is reduced, and fruit quality is affected. As the disease severity increases, the yield is reduced, mainly by the early drop of fruits from affected branches. The yield reduction can reach 30 to 100%, depending on proportion of affected canopy (8,11,22,57,91) (Fig. 36) and makes the orchard economically unfeasible in 7 to 10 years after planting (4,8,44,90). As disease severity increases, the percentage of affected fruit increases and can reach more than 40% (11,22). Those affected fruit are smaller, lighter, very acid, with reduced Brix, ratio, percentage of juice and soluble solids per box (11), and can affect the juice quality. Due to all those reasons, almost 100 million trees have been affected and destroyed in many countries of South and Southeast Asia, Indonesia, Philippines, India, Arabian Peninsula, and South Africa, compromising the local citriculture (9,13,47,104). Since 2004, more than 500 thousand trees were officially eliminated in Brazil due to HLB and it is estimated that an additional 300 to 400 thousand trees were unofficially eliminated by commercial citrus growers.
|
Fig. 36. Yield damage caused by HLB in 4 to 6 year-old sweet orange trees in São Paulo, Brazil. |
|
More quantitative information about the progress of disease incidence in the orchard, progress of severity in the tree canopy, and relationship between disease severity and yield, is necessary to develop economic models that can predicte the effect of disease in an endemic situation and evaluate the benefit of control measures over multiple years.
HLB Disease Control Strategies
With the finding that prokaryotic organisms are associated with HLB, efforts were made to control the disease by injecting trees with antibiotics (7,81,112), but only partial success was achieved and their use was abandoned (16). In South Africa, a management strategy was developed centering on production of disease-free budwood and nursery trees, psyllid control, and removal of infected trees. Details of current strategies were recently reviewed by Le Roux et al. (65) and reported also that HLB incidence has dropped from 38% in the 1960s to less than 1% presently (64).
In the late 1980s through early 1990s, UNDP, FAO conducted a multinational southeast Asian citrus rehabilitation project, the main goal of which was the greater understanding of HLB and development of practical disease control strategies (4). Perhaps the most important contribution of this project was the compilation of an overall strategy for HLB management based on multiple years of experience of Asian commercial producers and agricultural agencies with experience attempting control of HLB. The major recommendations resulting from this project are:
· geographical isolation and disease certification programs for budwood sources (mother trees);
· geographical isolation of nursery production;
· requirement that all citrus nursery production be conducted in secure insect proof screen houses;
· commercial plantation control of insect vectors by chemical insecticides and where applicable, via biocontrol to reduce HLB transmission; and
· commercial plantation removal of HLB infected trees to reduce HLB inoculum sources.
All of these recommendations require dedication of time, coordination among various segments of the industry, and carry financial burdens. Worldwide, few citrus industries have proactively taken the first three of these precautions prior to the introduction of HLB and/or other exotic pathogens and pests, and therefore are usually faced with the urgency of reacting to them post-introduction. The citrus industry of São Paulo Brazil was perhaps the best poised when HLB was discovered. This was because isolation of budwood sources and altered nursery production practices had been a necessity due to an onslaught of exotic diseases in Brazil such as citrus variegated chlorosis (CVC), citrus canker, severe isolates of citrus tristeza virus (CTV) stem pitting, leprosis virus, black spot, etc. When HLB was reported, Sao Paulo industry had already moved to budwood isolation, a nursery disease certification program, nursery industry isolation and regulation of the nursery industry requiring all nursery production be in secure insect-proof screenhouses. Florida was less well situated but has initiated changes throughout the citrus industry in response to HLB introduction. The Florida Department of Agriculture and Consumer Services has augmented their existing budwood nursery certification program with expanded testing for HLB and movement of certified budwood sources to an isolated greenhouse location in northern Florida outside of the commercial production area. The Florida nursery industry faces many challenges. Following a 10-year citrus canker eradication program that necessitated the removal of approximately 60% of the commercial nursery industry in an effort to eradicate the disease, the industry still remains largely a field production nursery industry and is locationally integrated within the boundaries of the commercial citrus production industry. The FDACS has required that all field-grown nursery production cease and that no field grown citrus nursery trees can be sold after January 2008. Additionally, all subsequent nursery production must be in insect-proof green houses or screen houses with a requisite 1-mi buffer where no HLB-infected commercial or residential citrus has been detected (per FDACS rule 5B-62 for budwood and nursery tree propagation). Thus the Florida citrus nursery industry is faced with massive restructuring and development of new infrastructure and possible acquisition of new land for nursery production outside of the commercial citrus area.
Chemical vector control. Perhaps the easiest HLB control strategy to accomplish is chemical control of HLB psyllid vectors. All commercial citrus production has access to the machinery or commercial grove care companies to accomplish chemical sprays for psyllid control, however the necessity of additional sprays is both costly and depending upon the economics of individual producers can be marginally feasible. Although there is much information on the effect of chemical control of various insecticides and programs on psyllid vectors, the effect of this practice on HLB increase and spread remains largely anecdotal and undocumented. An even greater challenge is the large population of HLB positive residential citrus trees where regulated chemical control is not an option.
Roguing as a control strategy. The effectiveness of roguing, or removal of diseased trees, as a control option is directly related to the latency of infection. The first occurrence of visual symptoms can be dramatic in some trees yet subtle in others. It is generally recommended that diseased trees should be removed but recognized that multiple asymptomatic but potentially infected trees, or trees with subclinical symptoms, probably exist in the vicinity. It is unknown how much inoculum these early stage infected trees, both asymptomatic and with limited symptoms, contribute for dispersal. Depending on the diligence and speed with which the individual grove manager removes trees after discovery, these early stage infections may contribute to more or less inoculum to the epidemic. From the discussion above we also know that many subclinical trees may also occur within an area surrounding individual infected trees. Roguing may be more effective if we take this subclinical portion of the population of infected trees into account and develop a threshold of tolerance that we will accept, beyond which entire blocks of commercial citrus are removed because of the estimated greater infection than apparent.
Regional prevalence. The incidence of HLB infections within the surrounding region greatly affects the probability and efficacy of slowing the epidemic. A commercial producer can be very diligent in vector control and rouging of infected trees, but if surrounding plantings are not as rigorously managed or large numbers of HLB-infected residential trees remain in the area, the planting may be overwhelmed with primary infections from surrounding infected trees and high vector populations. The efficacy of HLB control can be greatly increased by grower groups who take a regional approach and policy to HLB management. In addition, the efficacy of vector control and rouging is much higher in the initial stages of an epidemic. When HLB incidence builds up in areas over time, as it has in some places in Asia, the efficacy of even diligent HLB control efforts is much reduced.
Biological control of HLB vectors. The nymphs of both psyllid species are parasitized by hymenopterous ectoparasites Tamarixia dryi Waterston and T. radiatus Waterston, a fact that has been used to accomplish biological control of vector populations in Reunion Island and attempted elsewhere (3,19,24,29) (Figs. 37, 38, and 39). On Reunion Island, it is believed that the parasites significantly reduced the psyllid populations and lessened the damage of HLB. Elsewhere, biocontrol via introduction of parasites has also been attempted but has had very limited success (24). For instance, psyllid parasite biocontrol was attempted in Taiwan, but was not particularly effective. This is believed to be due to the presence of indigenous populations of hyperparasites that attack the hymenopterous ectoparasite biocontrol agents. Apparently these hyperparasites are not present on Reunion Island and therefore biocontrol has been more effective. Biological control is a topic of discussion for application in the western hemisphere as well. Field observation in Florida where T. radiatus was introduced, indicate that the effect of biological control can range broadly from 4 to 70% reduction in psyllid populations. Another internal parasitoid, Diaphorencyrtus aligarhensis, has also been found to attack D. citri (D. Hall unpublished observations). Unfortunately, the use of insecticides to reduce psyllid vectors of HLB also has a strong detrimental effect on biocontrol ectoparasite populations. What is not clearly understood, is the proportion of the psyllid population that must be controlled to have a significant effect on the rate of HLB increase and spread.
|
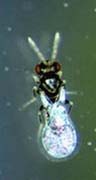 |
|
Fig. 37. Tetrasticus dryii, parasite and biocontrol agent of the Asian citrus psyllid, Diaphorina citri, the vector of Candidatus Liberibacter asiaticus, Ca. L. africanus, and presumably Ca. L americanus. |
|
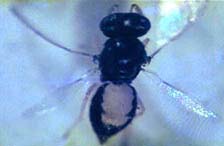 |
|
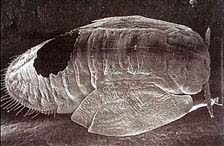 |
Fig. 38. Tamarixia radiata, parasite and biocontrol agent of the Asian citrus psyllid, Diaphorina citri, the vector of Candidatus Liberibacter asiaticus, Ca. L. africanus, and presumably Ca. L. americanus. |
|
Fig. 39. SEM of Asian citrus psyllid nymph parasitized by parasite T. dryii. Note hyperparasite exit hole in rear dorsal portion of nymph. Photo courtesy of Bernard Aubert. |
Novel control strategies. In late December of 2006, an international workshop on HLB was held in Ishigaki, Japan. At that meeting a collaborative project between the Vietnamese, Japanese, and Australian researchers was discussed and a potential new disease mitigating control strategy was discussed. The strategy is to interplant citrus with guava, which apparently inhibits the psyllid vector. This inhibition could be either direct via attracting the vector to the guava and killing it when it feeds presumably by some toxin, or indirectly by repelling or confusing the vector by some released volatile. The exact effect is unknown but preliminary data are encouraging (12). The effect of interplanting crops or mixed crops to deter insect pests and diseases via a “push-pull” strategy is not new, but rarely used in western agriculture (26,56,96,103). The potential of this strategy and its integration with more traditional management tools such as chemical vector control and roguing are being examined in commercial citrus plantings in Florida but are not recommended at this time due to insufficient research data. Also the use of high-density, short-term planting systems may have merit as a management strategy for HLB, to maximize early production and economic return, prior to an anticipated premature demise of a planting due to HLB.
Future Prognosis
There is little doubt that HLB is the most serious disease of citrus and that the commercial citrus industries in Florida and Brazil that are now dealing with this newly introduced disease are facing the toughest challenge they have yet faced. Other citrus producing areas and their commercial industries in the United States and Brazil have become highly sensitized to implications that an infection by HLB can have on their production, longevity, markets, and trade. This has led to the formation of task forces, research councils, and regulatory groups holding numerous discussions. The outcome has been the implementation of proactive survey and sampling methods to detect incipient infections, hopefully before they have an opportunity to spread and become endemic as well as immediate increases in research funding and pursuit of new funds to support research efforts in many areas related to HLB.
The consequences of HLB infection into those areas where it has already been introduced, and the prospect of more introductions into additional commercial citrus areas and states, are severe. Based on the experiences of other citrus producing countries, many changes quickly ensue within a newly infected commerical industry. In the short term, costly eradication, vector control, and nursery certification programs are immediately put in place. If nursery production cannot be maintained free of HLB, then insufficient numbers of replacement trees will exist for normal turnover combined with the increased demand due to increasing HLB-infected tree removals. This can result in a reduction of viable citrus production area as diseased trees are continuously removed. In addition, as individual trees become infected, the longevity of plantings decrease and, when they fall below a viable economic threshold, they must be removed and replanted. In some severly infected areas in Southeast Asia, this has resulted in orchard removal and replanting cycles as short as 3 to 5 years. In plantations practicing intensive HLB management, plantings can be sustained longer with vigilant vector control, tree removals, and replanting programs. However, this can result in heavy fiscal and manpower burdens. Invariably, many commercial growers cannot sustain the expense and move to alternative crops or prior citrus land succumbs to urbanization or other more fiscally beneficial land uses. This decline in citrus production will continue until it is eventually offset by higher profit-per-yield ratios driven by increased demand coupled with decreasing available and a new equilibrium is established composed of a smaller and more intensively managed industry.
In the future, improved early detection, maximal roguing strategies, better vector control, and novel new management strategies combined with economic threshold models to maximize rotation and profits, should lead to increase planting longevity and viability and a more viable and stable industry. In the long term, the answer to an endemic HLB situation is of course disease resistance. Unfortunately, no readily available source of resistance has yet been identified either for conventional breeding or transgenic improvement systems. However, it is hoped that efforts in pathogen and host sequencing and bioinformatics will point to resistance genes or pathogen vulnerabilities and thus paths for future incorporation of disease resistance mechanisms. At the present time, the citrus industry is composed of numerous commercial cultivars and species, the vast majority of which are moderately to highly susceptible to HLB. Thus when sources of resistance are identified, this resistance will have to be incorporated, perhaps using transgenic technology, into a large number of cultivars and species to meet with current marketing demands. Renovating such a complex industry as commerical citrus, will not be a trivial or short term undertaking.
Literature Cited
1. Aubert, B. 1987. Trioza erytreae Del Guercio and Diaphorina citri Kuwayama (Homoptera: Psylloidae), the two vectors of citrus greening disease: Biological aspects and possible control strategies. Fruits 42:149-162.
2. Aubert, B. 1987. Le greening, une maladie infectieuse des agrumes, d’origine bactérienne, transmise par des homoptères psyllides. Stratégie de lutte développée à l’île de la Réunion. Circonstances epidemiologigues en Afrique/Asie et modalités d’intervention. IRFA/CIAD-B. P. 180-97455 Saint Pierre Cedex.
3. Aubert, B., and Quilici, S. 1984. Biological control of psyllid vectors of greening disease in Reunion Island. Pages 118-123 in: Proc. of the 9th Conf. of the Intl. Organ. of Citrus Virologists. S. M. Garnsey, L. W. Timmer, and J. A. Dodds, eds. IOCV, Riverside, CA.
4. Aubert, B. 1990. Integrated activities for the control of huanglungbin-greening and its vector Diaphorina citri Kuwayama in Asia. Pages 133-144 in: B. Aubert, S. Tontyaporn, D. Buangsuwon,, eds., Rehabilitation of Citrus Industry in the Asia Pacific Region. Proc. of the Asia Pacific Intl. Conf. on Citriculture, Chiang Mai, Thailand , 4-10 February 1990. UNDP-FAO, Rome.
5. Aubert, B. 1992. Citrus greening disease, a serious limiting factor for citriculture in Asia and Africa. Proc. Intl. Soc. Citricult. 817-820.
6. Aubert, B., Grisoni, M., Villemin, M., and Rossolin, G. 1996. A case study of huanglongbing (greening) control in Reunion. Pages 276-278 in: Proc. of the 13th Conf. Intl. Organ. Citrus Virol. J. V. da Graça, P. Moreno, and R. K. Yokomi, eds. IOCV, Riverside CA.
7. Aubert, B., and Bové, J. M. 1980. Effect of penicillin or tetracycline injections of citrus trees affected by greening disease in Reunion Island. Pages 103-108 in: Proc. of the 8th Conf. of the Intl. Organ. of Citrus Virologists. E. C. Calavan, S. M. Garnsey, and L. W. Timmer, eds. IOCV, Riverside, CA.
8. Aubert, B., Sabine, A., Geslin, P., and Picardi, L. 1984. Epidemiology of the greening disease in Reunion Island before and after the biological control of the African and Asian citrus psyllas. Proc. Intl. Soc. Citricult. 1:440-442.
9. Aubert, B., Garnier, M., Guillaumin, D., Herbagyandodo, B., Setiobudi, L., and Nurhadi, F. 1985. Greening, a serious disease threat for the citrus production of the Indonesian archipelago. Future prospects of integrated control. Fruits 40:549-563.
10. Bassanezi, R. B., Busato, L. A., Bergamin Filho, A., Amorim, L., and Gottwald, T. R. 2005. Preliminary spatial pattern analysis of Huanglongbing in São Paulo, Brazil. Pages 341-355 in: Proc. of the 16th Conf. of the Intl. Organ. of Citrus Virologists. M. E. Hilf, N. Duran-Vila, and M. A. Rocha-Peña. IOCV, Riverside, CA.
11. Bassanezi, R. B., Montesino, L. H., Busato, L. A. and Stuchi, E. S. 2006. Damages caused by huanglongbing on sweet orange yield and quality in São Paulo. Page 39 in: Proc. of the Huanglongbing-Greening Intl. Workshop, Ribeirão Preto, Brazil.
12. Beattie, G. A. C., Holford, P., Mabberley, D. J., Haigh, A. M., Bayer, R., and Broadbent, P. 2006. Aspects and insights of Australia-Asia collaborative research on Huanglongbing. Pages 47-64 in: Proc. of the Intl. Workshop for the Prevention of Citrus Greening Disease in Severely Infected Areas. Intl. Res. Div., Agric. Forestry Fisheries Res. Counc. Secretariat, Ministry of Agric., Forestry and Fisheries, Tokyo, Japan.
13. Bové, J. M. 1986. Greening in Arab Peninsula: Towards new techniques for its detection and control. FAO Plant Prot. Bull. 34:7-14.
14. Bové, J. M. 2006. Huanglongbing: A destructive, newly-emerging, century-old disease of citrus. J. Plant Pathol. 88:7-37.
15. Bové, J. M., Erti Dwiastuti, M., Triviratno, A., Supriyanto, A., Nasli, E., Becu, P., and Garnier, M. 2000. Incidence of huanglongbing and citrus rehabilitation in North Bali, Indonesia. Pages 200-206 in: Proc. of the 14th Intl. Organ. Citrus Virol. J. V. da Graça, R. F. Lee, and R. K. Yokomi, eds. IOCV Riverside, CA.,
16. Buitendag, C. H., and von Broembsen, L. A. 2003. Living with citrus greening disease. Pages 269-271 in: Proc. of the 12th Conf. Intl. Organ. Citrus Virol. P. Moreno, J. V. da Graça and L. W. Timmer, eds. IOCV, Riverside, CA.
17. Capoor, S. P. 1963. Decline of citrus trees in India. Bull. Nat. Inst. Sci. India 24:48-64.
18. Capoor, S. P., Rao, D. G., and Viswanath, S. M. 1967. Diaphorina citri Kuway, a vector of the greening disease of citrus in India. Ind. J. Agric. Sci. 37:572-576.
19. Catling, H. D. 1969. The bionomics of the South African citrus psylla Trioza erytreae Del Guercio (Homoptera: Psyllidae). 2. The influence of parasites and notes on the main species involved. J. Entomol. Soc. South. Afr. 32:209-223.
20. Catling, H. D. 1969. The bionomics of the South African psylla, Trioza erytreae Del Guercio (Homoptera: Psyllidae). 3. The influence of extremes of weather on survival. J. Entomol. Soc. S. Afr. 32:273-290.
21. Catling, H. D. 1970. The bionomics of the South African citrus psylla, Trioza erytreae Del Guercio (Homoptera:Psyllidae). 4. Influence of predators. J. Entomol. Soc. S. Afr. 33:341-348.
22. Catling, H. D., and Atkinson, P. R. 1974. Spread of greening by Trioza erytreae (Del Guercio) in Swaziland. Pages 33-39 in: Proc. of the 6th Conf. Intl. Organ. Citrus Virologists. L. G. Weathers and M. Cohen, eds. IOCV, Div. Agric. Sci., Univ. of Calif., Riverside.
23. Chao, H. Y., Chiang, Y. H., Lee, S. L., Chiu, C. S., and Su, W. F. 1979. A preliminary study on the relation between the prevalence of the Citrus Yellow Shoot (Huanglungbin) and the citrus psyllid Diaphorina citri Kuwayama. Acta Phytopathol. Sin. 2:121-126.
24. Chiu, S.-C., Aubert, B., and Chien, C. C. 1988. Attempts to establish Tetrastichus radiatus Waterston in Taiwan. Pages 265-268 in: Proc. of the 10th Conf. Intl. Organ. Citrus Virologists. L. W. Timmer, S. M. Garnsey, and L. Navarro, eds. IOCV, Riverside, CA.
25. Colleta-Filho, H. D., Targon, M. L. P. N., Takita, M. A., De Negri, J. D., Amaral, A. M., Müller, G. W., Pompeu Júnior, J., Carvalho, S. A., and Machado, M. A. 2004. Detecção do agente causal do greening do citros (Candidatus Liberibacter asiaticus) no estado de São Paulo. Summa Phytopathol. 30:510.
26. Cook, S. M., Zeyaur, R. K., and Pickett, J. A. 2007. The use of Push-Pull strategies in integrated pest management. Annu. Rev. Entomol. 52:375-400.
27. da Graça, J. V. 1991. Citrus greening disease. Annu. Rev. Phytopathol. 29:109-136.
28. da Graça, J. V., and Korsten, L. 2004. Citrus huanglongbing: Review, present status and future strategies. Pages 229-245 in: Diseases of Fruits and Vegetables Vol. I (S. A. M. H. Naqvi, ed.). Kluwer Acad. Press, Dordrecht.
29. Etienne, J., and Aubert, B. 1980. Biological control of psyllid vectors of greening disease in Reunion Island. Pages 118-121 in: Proc. of the 8th Conf. Intl. Organ. Citrus Virologists. E. C. Calavan, S. M. Garnsey, and L. W. Timmer, eds. IOCV, Riverside, CA.
30. Fraser, L. R., Singh, D., Capoor, S. P., and Nariani, T. K. 1966. Greening virus, the likely cause of citrus dieback in India. FAO Plant Prot. Bull. 14:127-130.
31. French, J. V., Kahlke, C. J., and da Graça, J. V. 2001. First record of the Asian citrus psylla, Diaphorina citri Kuwayama (Homoptera: Psyllidae), in Texas. Subtrop. Plant Sci. 53:14-15.
32. Garnier, M., and Bové, J. M. 1978. The organism associated with citrus greening disease is probably a member of the schizomycetes. Zentralbl. Bakteriol. Parasitenkd. Infektions, Kra. Hyg. Reihe A 241:221-222.
33. Garnier, M., and Bové, J. M. 1993. Citrus greening disease and the greening bacterium. Pages 212-219 in: Proc. of the 12th Conf. Intl. Organ. Citrus Virologists. P. Moreno, J. V. da Graça, and L. W. Timmer, eds. IOCV, Riverside, CA.
34. Garnier, M., Danel, N., and Bové, J. M. 1984. Etiology of citrus greening disease. Ann. Microbiol. (Inst. Pasteur) 135A:169-179.
35. Garnier, M., Jagoueix-Eveillard, S., Cronje, C. P. R., Le Roux, H. F., and Bové, J. M. 2000. Genomic characterization of a liberibacter present in an ornamental rutaceous tree, Calodendron capense in the Western Cape province of South Africa. Intl. J. Syst. Evol. Microbiol. 50:2119-2125.
36. Garnier, M., Martin-Gros, G., and Bové, J. M. 1987. Monoclonal antibodies against the bacterial-like organism associated with citrus greening disease. Ann. Inst. Pasteur (Microbiol.) 138:639-650.
37. Gatineau, F., Loc, H. T., Tuyen, N. D., Tuan, T. M., Hien, N. T. D., and Truc, N. T. N. 2006. Effects of two insecticide practices on population dynamics of Diaphorina citri and huanglongbing incidence in south Vietnam. Page 110 in: Proc. of the Huanglongbing–Greening Intl. Workshop, Ribeirão Preto, Brazil.
38. Gottwald, T. R., Aubert, B., and Huang, K. L. 1991. Spatial pattern analysis of citrus greening in Shantou, China. Pages 421-427 in: Proc. of the 11th Conf. Intl. Org. Citrus Virol. R. H. Brlansky, R. F. Lee, and L. W. Timmer, eds. IOCV, Riverside, CA.
39. Gottwald. T. R., Aubert, B., and Zhao, X.-Y. 1989. Preliminary analysis of citrus greening (Huanglungbin) epidemics in the People's Republic of China and French Reunion Island. Phytopathology 79:687-693.
40. Gottwald T. R., Bergamin-Filho, A., Bassanezi, R. B., Amorim, L., Irey, M., Zhao, X., and Aubert, B. 2006. Concepts in Huanglongbing Epidemiology. Pages 1-10 in: Proc. of the Intl. Workshop for the Prevention of Citrus Greening Disease in Severely Infected Areas. Intl. Res. Div., Agric. Forestry Fisheries Res. Counc. Secretariat, Ministry of Agric., Forestry and Fisheries, Tokyo, Japan.
41. Gottwald, T. R., Cambra, M., Moreno, P., Camarasa, E., and Piquer, J. 1996. Spatial and temporal analyses of citrus tristeza in Eastern Spain. Phytopathology 86:45-55.
42. Gottwald, T. R., Garnsey, S. M., and Borbón, J. 1998. Increase and patterns of spread of citrus tristeza virus infections in Costa Rica and the Dominican Republic in the presence of the brown citrus aphid, Toxoptera citricida. Phytopathology 88:621-636.
43. Gottwald, T. R., Gibson, G., Garnsey, S. M., and Irey, M. 1999. Examination of the effect of aphid vector population composition on the spatial dynamics of citrus tristeza virus spread via stochastic modeling. Phytopathology 89:603-608.
44. Gottwald, T. R., Gonzales, C. I., and Mercado, B. G. 1991. Analysis of the distribution of citrus greening in groves in the Philippines. Pages 414-420 in: Proc. of the 11th Conf. Intl. Org. Citrus Virol. R. H. Brlansky, R. F. Lee, and L. W. Timmer, eds. IOCV, Riverside, CA.
45. Gottwald, T. R., Richie, S. M., and Campbell, C. L. 1992. LCOR2 - Spatial correlation analysis software for the personal computer. Plant Dis. 76:213-215.
46. Halbert, S. 2005. The discovery of huanglongbing in Florida. Page 50 in: Proc. of the 2nd Intl. Citrus Canker and Huanglongbing Worksh., Orlando, FL.
47. Halbert, S. E., and Manjunath, K. L. 2004. Asian citrus psyllids (Sternorrhyncha: Psyllidae) and greening disease in citrus: A literature review and assessment of risk in Florida. Fla. Entomol. 87:330-354.
48. Halbert, S. E., and Nuñez, C. A. 2004. Distribution of the Asian citrus psyllid, Diaphorina citri Kuwayama (Sternorrhyncha: Psyllidae) in the Caribbean basin. Fla. Entomol. 87:401-402.
49. Husain, M. A., and Nath, D. 1927. The citrus psylla (Diaphorina citri Kuw.)[Psyllidae: Homoptera]. Mem. Dept. Agric. India, Entomol. Ser. 10:1-27.
50. Hughes, G., and Madden, L. V. 1992. Aggregation and incidence of disease. Plant Pathol. 41:657-660.
51. Hughes, G., and Madden, L. V. 1993. Using the beta-binomial distribution to describe aggregated patterns of disease incidence. Phytopathology 83:759-763.
52. Hughes, G., McRoberts, N., Madden, L. V., and Gottwald, T. R. 1997. Relationships between disease incidence at two levels in a spatial hierarchy. Phytopathology 87:542-550.
53. Hung, T.-H., Wu, M.-L., and Su, H.-J. 2000. Identification of alternative hosts of the fastidious bacterium causing citrus greening disease. J. Phytopathol. 148:321-326.
54. Hung, T.-H., Wu, M.-L., and Su, H.-J. 2001. Identification of the Chinese box orange (Severinia buxifolia) as an alternative host of the bacterium causing citrus huanglongbing. Eur. J. Plant Pathol. 107:183-189.
55. Irey, M. S., Gast, T., and Gottwald, T. R. 2006. Comparison of visual assessment and polymerase chain reaction assay testing to estimate the incidence of the Huanglongbing pathogen in commercial Florida citrus. Pages 88-93 in: Proc. of the Fla. State Hortic. Soc.
56. Isman, M. B. 2006. Botanical insecticides, deterrents, and repellents in modern agriculture and an increasingly regulated world. Annu. Rev. Entomol. 51:45–66.
57. Ke, S., Li, K. B., Ke, C., and Tsai, J. H. 1988. Transmission of the huanglungbin agent from citrus to periwinkle by dodder. Pages 258-264 in: Proc. of the 10th Conf. Intl. Organ. Citrus Virol. L. W. Timmer, S. M. Garnsey, and L. Navarro, eds. IOCV, Riverside, CA.
58. Knapp, J., Halbert, S., Lee, R., Hoy, M., Clark, R., and Kesinger, M. 1998. The Asian psyllid and citrus greening disease. Citrus Ind. 79:28-29.
59. Korsten, L., Sanders, G. M., Su, H. J., Garnier, M., Bové, J. M., and Kotzé, J. M. 1993. Detection of citrus greening-infected citrus in South Africa using a DNA probe and monoclonal antibodies. Pages 224-232 in: Proc. of the 12th Conf. Intl. Organ. Citrus Virol. P. Moreno, J. V. da Graça, and L. W. Timmer, eds. IOCV, Riverside, CA.
60. Korsten, L., Jagoueix, S., Bové, J. M., and Garnier, M. 1996. Huanglongbing (greening) detection in South Africa. Pages 395-398 in: Proc. of the 13th Conf. Intl. Organ. Citrus Virol. J. V. da Graça, P. Moreno, and R. K. Yokomi, eds. IOCV, Riverside, CA.
61. Laflèche, D., and Bové, J. M. 1970. Structures de type mycoplasme dans les feuilles d’orangers atteints de la maladie du greening. C. R. Acad. Sci. Ser. D 270:455-465.
62. Lallemand, J., Fos, A., and Bové, J. M. 1986. Transmission de la bacterie associée à la forme africaine de la maladie du “greening’ par le psylle asiatique Diaphorina citri Kuwayama. Fruits 41:341-343.
63. Lee, H. A. 1921. The relation of stocks to mottled leaf of citrus leaves. Phil. J. Sci. 18:85-95.
64. Le Roux, H. F., van Vuuren, S. P., and Manicom, B. Q. 2006. Huanglongbing in South Africa. Pages 5-9 in: Proc. of the Huanglongbing-Greening Intl. Workshop, Ribeirão Preto, Brazil.
65. Le Roux, H. F., van Vuuren, S. P., Pretorius, M. C., and Buitendag, C. H. 2006. Management of huanglongbing in South Africa. Pages 43-47 in: Proc. of the Huanglongbing-Greening Intl. Workshop, Ribeirão, Brazil.
66. Lin, C. K. 1963. Notes on citrus yellow shoot disease. Acta Phytophylact. Sin. 2:243-251.
67. Lin, K. H. 1956. Observations on yellow shoot on citrus. Etiological studies of yellow shoot of citrus. Acta Phytopathol. Sin. 2:237-242.
68. Lopes, S. A., Martins, E. C., and Frare, G. F. 2005. Detecção de Candidatus Liberibacter americanus em Murraya paniculata. Summa Phytopathol. 31:48-49.
69. Lopes, S. A., Martins, E. C., and Frare, G. F. 2006. Detecção de Candidatus Liberibacter asiaticus em Murraya paniculata. Fitopatol. Bras. 31:303.
70. Lopes, S. A., Frare, G. F., and Martins, E. C. 2006. Hosts of liberibacter in Brazil. Page 5 in: Proc. of the Huanglongbing-Greening Intl. Workshop, Ribeirão Preto, Brazil.
71. Lopes, S. A., Frare, G. F., Yamamoto, P. T., and Ayres, A. J. 2006. Inefficacy of pruning to control citrus huanglongbing in Brazil. Page 49 in: Proc. of the Huanglongbing–Greening Intl. Workshop, Ribeirão Preto, Brazil.
72. Madden, L. V., and Hughes, G. 1994. BBD – Computer software for fitting the beta-binomial distribution to disease incidence data. Plant Dis. 78:536-540.
73. Madden, L. V., Louie, R., Abt, J. J., and Knoke, J. K. 1982. Evaluation of tests for randomness of infected plants. Phytopathology 72:195-198.
74. Manicom, B. Q., and van Vuuren, S. P. 1990. Symptoms of greening disease with special emphasis on African greening. Pages 127-131 in: Proc. of the 4th Intl. Asia-Pacific Conf. Citrus Rehabilitation, Chang Mai, Thailand.
75. Martinez, A. L., and Wallace, J. M. 1967. Citrus leaf-mottle-yellow disease in the Philippines and transmission of the causal virus by a psyllid, Diaphorina citri. Plant Dis. Rep. 51:692-695.
76. Massonie, G., Garnier, M., and Bové, J. M. 1976. Transmission of Indian greening by Trioza erytreae (Del Guercio), the vector of South African greening. Pages 18-20 in: Proc. of the 7th Conf. Intl. Organ. Citrus Virol. E. C. Calavan, ed. IOCV, Riverside, CA.
77. McClean, A. P. D., and Oberholzer, P. C. J. 1965. Citrus psylla, a vector of greening disease of sweet orange. S. Afr. J. Agric. Sci. 8:297-298.
78. McClean, A. P. D., and Oberholzer, P. C. J. 1965. Greening disease of sweet orange: Evidence that it is caused by a transmissible virus. S. Afr. J. Agric. Sci. 8:253-276.
79. McClean, A. P. D., and Schwarz, R. E. 1970. Greening or blotchy-mottle disease of citrus. Phytophylactica 2:177-194.
80. Modjeska, J. S., and Rawlings, J. O. 1983. Spatial correlation analysis of uniformity data. Biometrics 39:373-384.
81. Moll, J. N., and van Vuuren, S. P. 1977. Greening disease in Africa. Prot. Intl. Soc. Citricult. 3:903-912.
82. Moreno, P., da Graça, J. V., and Yokomi, R. K. 1996. Preface. Pages v-vi in: Proc. of the 13th Conf. Intl. Organ. Citrus Virol. P. Moreno, J. V. da Graça, and R. K. Yokomi, eds. IOCV, Riverside, CA.
83. Nariani, T. K., Raychaudhuri, S. P., and Viswanath, S. M. 1973. Tolerance to greening in certain citrus species. Curr. Sci. 42:513-514.
84. Oberholzer, P. C. J., von Standen, D. F. A., and Basson, W. J. 1965. Greening disease of sweet orange in South Africa. Pages 213-219 in: Proc. of the 3rd Conf. Intl. Organ. Citrus Virol. W. C. Price, ed. Univ. Florida Press, Gainesville, FL.
85. Ôtake, A. 1990. Bibliography of citrus greening disease and its vectors attached with indices, and a critical review on the ecology of the vectors and their control. Jap. Intl. Coop. Agency.
86. Raychaudhuri, S. P., Nariani, T. K., and Lele, V. C. 1969. Citrus die-back problem in India. Proc. of the 1st Intl. Citrus Symp. 3:1433-1437.
87. Raychaudhuri, S. P., Nariani, T. K., Ghosh, S. K., Viswanath, S. M., and Kumar, D. 1974. Recent studies on citrus greening in India. Pages 53-57 in: Proc. of the 6th Conf. Intl. Organ. Citrus Virol. L. G. Weathers and M. Cohen, eds. Univ. California, Div. Agric. Sci.
88. Regmi, C., and Lama, T. K. 1987. Greening incidence and greening vector population dynamics in Pokhara. Pages 238-243 in: Proc. of the 10th Conf. Intl. Organ. Citrus Virol. L. W. Timmer, S. M. Garnsey, and L. Navarro, eds. IOCV, Riverside, CA.
89. Reinking, O. A. 1919. Diseases of economic plants in southern China. Phil. Agric. 8:109-135.
90. Roistacher, C. N. 1996. The economics of living with citrus diseases : huanglongbing (greening) in Thailand. Pages 279-285 in: Proc. of the 13th Conf. Intl. Organ. Citrus Virol. P. Moreno, J. V. da Graça, and R. K. Yokomi, eds. IOCV, Riverside, CA.
91. Schwarz, R. E. 1967. Results of a greening survey on sweet orange in the major citrus growing areas of the Republic of South Africa. S. Afr. J. Agric. Sci. 10:471-476.
92. Schwarz, R. E. 1968. Greening disease. Pages 87-90 in: Indexing Procedures for 15 Virus Diseases of Citrus. USDA ARS Agric. Handbook No. 333.
93. Schwarz, R. E. 1968. Indexing of greening and exocortis through fluorescent marker substances. Pages 118-124 in: Proc. of the 4th Conf. Intl. Organ. Citrus Virol. J. F. L. Childs, ed. IOCV, Riverside, CA.
94. Schwarz, R. E. 1968. Thin layer chromatographical studies on phenol markers of the greening virus in various citrus species. S. Afr. J. Agric. Sci. 11:797-801.
95. Schwarz, R. E., Knorr, L. C., and Prommintara, M. 1973. Presence of citrus greening and its psylla vector in Thailand. FAO Plant Prot. Bull. 21:132-138,
96. Shelton A. M., and Badenes-Perez, F. R. 2006. Concepts and applications of trap cropping in pest management. Annu. Rev. Entomol. 51:285-308.
97. Su, H. J., and Wu, R. Y. 1979. Preliminary study on the etiology of Wentan pomelo decline. Proc. of the ROC-UIS Co-op. Sci. Sem. Mycoplasma Dis. Plants (Nat. Sci. Council Symp. Ser. No.1): 45-57.
98. Sutton, B. D., Duan, Y.-P., Halbert, S., Sun, X.-A., Schubert, T., and Dixon, W. 2005. Detection and identification of citrus huanglongbing (greening) in Florida, USA. Page 59 in: Proc. of the 2nd Intl. Citrus Canker and Huanglongbing Workshop, Orlando, FL.
99. Teixeira, D. C., Ayres, A. J., Kitajima, E. W., Tanaka, F. A. O., Danet, J. L., Jagoueix-Eveillard, S., Saillard, C., and Bové, J. M. 2005a. First report of a Huanglongbing-like disease of citrus in São Paulo State, Brazil, and association of a new liberibacter species, "Candidatus Liberibacter americanus", with the disease. Plant Dis. 89:107.
100. Teixeira, D. C., Saillard, C., Jagoueix-Eveillard, S., Danet, J. L., Ayres, A. J., and Bové, J. M. 2005b. "Candidatus Liberibacter americanus" associated with citrus huanglongbing (greening disease) in São Paulo State, Brazil. Intl. J. Syst. Evol. Microbiol. 55:1857-1862.
101. Teixeira, D. C., Danet, J. L., Eveillard, S., Martins, E. C., Jesus Junior, W. C., Yamamoto, P. T., Lopes, S. A., Bassanezi, R. B., Ayres, A. J., Saillard, C., and Bové, J. M. 2005. Citrus huanglongbing in São Paulo State, Brazil: PCR detection of the “Candidatus’ Liberibacter species associated with the disease. Mol. Cell. Probes 19:173-179.
102. Tirtawidjaja, S. 1980. Citrus virus research in Indonesia. Pages 129-132 in: Proc. of the 5th Conf. Intl. Organ. Citrus Virol. W. C. Price, ed. Univ. Florida Press, Gainesville, FL.
103. Tonhasca, A., and Byrn, D. N. 1994. The effects of crop diversification on herbivorous insects: A meta-analysis approach. Ecol. Entomol. 19:239-244.
104. Toorawa, P. 1998. La maladie du huanglongbing (greening) des agrumes a l’île Maurice. Detection de "Candidatus Liberobacter asiaticum" et "Candidatus Liberobacter africanum" dans les agrumes et les insects vecteurs. Doctoral Thesis, L’Université de Bordeaux.
105. Van den Berg, M. A., van Vuuren, S. P., and Deacon, V. E. 1991-92. Studies on greening disease transmission by the citrus psylla, Trioza erytreae (Hemiptera: Triozidae). Israel J. Entomol. 25-26:51-56.
106. Van der Merwe, A. J., and Andersen, F. G. 1937. Chromium and manganese toxicity. Is it important in Transvaal citrus greening? Fmg. S. Afr. 12:439-440.
107. Weinert, M. P., Jackson, S. C., Grimshaw, J. F., Bellis, G. A., Stephens, P. M., Gunua, T. G., Kame, M. F., and Davis, R. I. 2004. Detection of Huanglongbing (citrus greening disease) in Timor-Leste (East Timor) and in Papua New Guinea. Aust. Plant Pathol. 33:135-136.
108. Wulff, N. A., Teixeira, D. C., Martins, E. C., Leite, A. P. R., Padiar, N. R. H., Mariano, A. G., Carmo, A. E., Abrahão, D. P., Sousa, M. C., Ayres, A. J., and Bové, J. M. 2006. Huanglongbing diagnosis. Page 17 in: Proc. of the Huanglongbing-Greening Intl. Workshop, Ribeirão Preto, Brazil.
109. Xu, C. F., Xia, Y. H., Li, K. B., and Ke, C. 1988. Further study of the transmission of citrus huanglongbing by a psyllid, Diaphorina citri Kuwayama. Pages 243-248 in: Proc. of the 10th Conf. Intl. Organ. Citrus Virol. L. W. Timmer, S. M. Garnsey, and L. Navarro, eds. IOCV, Riverside, CA.
110. Yamamoto, P. T., Felippe, M. R., Garbim, L. F., Coelho, J. H. C., Ximenes, N. L., Martins, E. C., Leite, A. P. R., Sousa, M. C., Abrahão, D. P., and Braz, J. D. 2006. Diaphorina citri (Kuwayama) (Hemiptera: Psyllidae): vector of the bacterium Candidatus Liberibacter americanus. Page 96 in: Proc. of the Huanglongbing-Greening Intl. Workshop, Ribeirão Preto, Brazil.
111. Zhang, W. C. 1981. Development and outlook of citrus industry in China. Proc. of the Intl. Soc. Citricult. 2:987-990.
112. Zhao, X.-Y. 1981. Citrus yellow shoot (Huanglungbin) in China: A review. Proc. of the Intl. Soc. Citricult. 1:466-469.
113. Zhao X.-Y. 2006. Huanglongbing in China. Page 3 in: Proc. of the Huanglongbing-Greening Intl. Workshop, Ribeirão Preto, Brazil.