The Burkholderia cepacia complex (Bcc) is a group of at least 9 closely related species (often called “genomovars”) that is currently attracting considerable attention for their extraordinary versatility as plant pathogens, saprophytes, biocontrol agents, bioremediation agents, and human pathogens.
Formerly known as Pseudomonas cepacia, the bacterium now known as Burkholderia cepacia, was first described in 1950 as the cause of sour skin of onions by Cornell University plant pathologist Walter Burkholder (1). Subsequently, P. cepacia was renamed Burkholderia cepacia (2) and transferred to the beta subdivision of the proteobacteria (3).
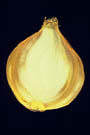
|
B. cepacia complex bacteria as phytopathogens.
Click the image to see an enlarged view.
Click here to go to the
Sour Skin section from the
Compendium of Onion and Garlic Diseases, APS PRESS. |
|
B. cepacia complex bacteria as biocontrol agents. Click the image to see an enlarged view.
Click here to see other related
B. cepacia biocontrol articles from APS Journals. |
B. cepacia complex bacteria as biocontrol agents
B. cepacia complex bacteria are naturally abundant in soil, water, and on plant surfaces (4). They are distinctive in their ability to metabolize a broad range of organic compounds as carbon and energy sources, an attribute which has spurred the development of their use in bioremediation of soil and groundwater contaminated with chlorinated hydrocarbons (5) and herbicides (6). B. cepacia complex organisms have also been the focus of considerable research by plant pathologists who have shown that some strains are effective biocontrol agents against soilborne (7-11), foliar (12), and post-harvest diseases (13-15). Many strains of the B. cepacia complex produce one or more antibiotics active against a broad range of plant pathogenic fungi (16,17). These antibiotics appear, in many cases, to be important for disease suppression. Biocontrol with this group of organisms can be an effective substitute for chemical pesticides which may pose risks to human health and the environment. Several B. cepacia complex strains have in the past been registered by the U.S. EPA for use as microbial pesticides (biological control agents). The products include Blue Circle and Deny (Stine Microbial Products).
|
B. cepacia complex bacteria as human pathogens. (Photo courtesy J. Govan)
Click the image to see an enlarged view.
|
B. cepacia complex bacteria as human pathogens
Some scientists are concerned about the use of B. cepacia complex bacteria for biocontrol because certain strains are opportunistic human pathogens. B. cepacia complex organisms are responsible for some nosocomial (hospital-derived) infections, and most alarmingly, some strains can cause fatal lung infection of individuals with cystic fibrosis (CF) (18). Antibiotic treatment is confounded by the organisms’ resistance to multiple antibiotics. Cystic fibrosis results from a genetic defect in sodium and chloride transport within epithelial cells, leading to abnormally thick mucus accumulation in the lungs. The mucus clogs the lungs, predisposing patients to chronic and eventually fatal infections by a succession of bacteria including Pseudomonas aeruginosa. (For more information about cystic fibrosis, see the
Cystic Fibrosis Foundation webpage. In the early 1980s Burkholderia cepaciabecame associated with epidemics of severe lung infections ("cepacia syndrome") traced, in many cases, to CF treatment centers and social gatherings where CF patients apparently acquired the disease from other B. cepacia-infected individuals (19).
The environment as a source of B. cepacia complex infections
Indirect evidence for the speculation that the environment serves as a reservoir for acquisition of novel B. cepacia complex species comes from the observation that infection control measures (including segregation of patients) have reduced but not eliminated new infections. Direct evidence was obtained from genotyping studies, in which clinical and environmental isolates were compared using state-of-the-art molecular fingerprinting techniques. For example, it was shown that the B. cepacia type strain ATCC 25416T (isolated from rotting onions in the 1940s) was also isolated from sputum of a CF patient in the UK (32). Similalrly, it was reported that B. cenocepacia strain PHDC recovered from most CF patients in the mid-Atlantic region of the USA could also be isolated from agricultural soils in the same region (32). Similarly, based on molecular fingerprinting, B. ambifaria (genomovar VI) strain AMMD, a well-characterized biocontrol isolate was almost identical to a strain recovered from a cystic fibrosis patient. Interestingly, both strains had the same geographic origin, Wisconsin, even though their sources were distinct, soil and cystic fibrosis sputum (33). These findings, although anecdotal, show that human isolates are not necessarily distinct from environmental ones.
Identifying strains pathogenic to humans
Chief among the concerns is distinguishing between human pathogenic and non-pathogenic strains of B. cepacia. Numerous studies have attempted to separate these strains on the basis of pectinase production, pathogenicity to onion, bacteriocin production and susceptibility, isozyme profiles, and genomic analysis using PFGE and RAPDs (20-25). A molecular taxonomic approach has been developed for differentiating among the heterogeneous group of strains identified as B. cepacia (26,27). They and others (28,29, 34) have shown that the B. cepacia complex is comprised of at least 9 distinct genomovars/species. Most of the highly transmissible CF strains belong to genomovar III (recently renamed as Burkholderia cenocepacia) (35), whereas most of the strains from non-clinical sources belong to genomovars I (= B. cepacia sensu stricto), V (= B. vietnamiensis), and VII (B. dolosa). However, strains representing all genomovars have been isolated from CF patients and environmental samples (32). Genetic markers have been developed to detect certain highly transmissible CF strains using probes for the cable pilin gene, cblA (30) or for a 1.4 kb fragment called BCESM (B. cepacia epidemic strain marker) (31). While these methods are valuable for rapid detection of certain epidemic strains, they are not useful for clearly distinguishing human pathogenic vs. non-pathogenic strains. Identification of virulence factors required for human infection and the development of molecular probes to detect them would aid greatly in reliable determination of risk associated with individual B. cepacia complex strains. Genomic sequencing of B. cepacia complex strains could prove extremely useful in this regard, and currently several of these projects are underway (for an overview,
see http://users.ugent.be/~tcoenye/index_bestanden/index.htm).
Regulatory issues
The EPA has proceeded cautiously on applications for experimental use permits or registration of new B. cepacia strains for biocontrol because of the uncertainties regarding its potential risks (
click here for the Phyto News story, “EPA Notice on Microbial Pesticides Concerns”). In 1999, a scientific advisory panel was convened by EPA to consider the risk associated with the use of B. cepacia as a microbial pesticide. In 2002 the EPA proposed a significant new use rule (SNUR) under section 5(a)(2) of the Toxic Substances Control Act (TSCA) for the Burkholderia cepacia complex. This proposed rule would require persons who intend to manufacture, import, or process Bcc for a significant new use to notify EPA at least 90 days before commencing the manufacturing(including import) or processing of Bcc for a use designated by this SNUR as a significant new use. The required notice would provide EPA with the opportunity to evaluate the intended new use and associated activities and, if necessary, to prohibit or limit that activity before it occurs. In 2004, EPA issued a final rule
(http://www.epa.gov/fedrgstr/EPA-PEST/2004/September/Day-29/p21695.htm), revoking all tolerances for the B. cepacia complex, because EPA deleted food uses from registrations following requests for voluntary cancellation or use deletion by the registrants. This means that at present it is not possible to use these organisms as biocontrol agents.
Establishing a dialogue
APS has recognized the need to establish a scientific dialogue about B. cepacia complex among plant pathologists, medical microbiologists and representatives from regulatory agencies. A symposium, "Burkholderia cepacia: Friend or Foe?", sponsored by the APS Biological Control Committee, was held at the 1998 APS Annual Meetings in Las Vegas on Nov. 9. (
Click here to see the symposium agenda.) International experts offered their insights on the biology, epidemiology, and genetics of this fascinating organism with the goal of developing a knowledge-based approach to evaluating potential risks associated with the use of B. cepacia complex in agriculture. Plant pathologists now participate in annual meetings of the International Burkholderia cepacia Working Group (
see http://users.ugent.be/~tcoenye/index_bestanden/index.htm for more information) and are actively engaged in joint research projects with medical researchers to help unravel the the mysteries of this fascinating group of organisms.
References
1. Burkholder,W. 1950. Sour skin, a bacterial rot of onion bulbs. Phytopathology 40:115-118.
2. Yabuuchi, E. Kosako, Y., Oyaizu, H., Yano, I., Hotta, H., Hashimoto, Y. Ezaki, T., and Arakawa, M. 1992. Proposal of
Burkholderia gen. nov. and transfer of seven species of the genus
Pseudomonas homology group II to the new genus, with the type species
Burkholderia cepacia (Palleroni and Holmes, 1981) comb. nov. Microbiol. Immunol. 36:1251-1275.
3. Olsen, G. J., Woese, C. R., and Overbeek, R. 1994. The winds of (evolutionary) change: breathing new life into microbiology. J. Bacteriol. 176:1-6.
4. McArthur, J. V., Kovacic, D. A., and Smith, M. H. 1988. Genetic diversity in natural populations of a soil bacterium across a landscape gradient. Proc. Natl. Acad. Sci. USA 85:9621-9624.
5. Krumme, M. L. Timmis, K. N., and Dwyer, D. F. 1993. Degradation of trichloroethylene by
Pseudomonas cepacia G4 and the constitutive mutant strain G4 5223 PR1 in aquifer microcosms. Appl. Environ. Microbiol. 59:2746-2749.
6. Sangodkar, U. Chapman, P., Chakrabarty, A. 1988. Cloning, physical mapping and expression of chromosomal genes specifiying degradation of the herbicide 2,4,5-T by
Pseudomonas cepacia AC1100. Gene 71:267-277.
7. McLoughlin, T.J. Quinn, J.P. Bettermann, A. Bookland, R. 1992.
Pseudomonas cepacia suppression of sunflower wilt fungus and role of antifungal compounds in controlling the disease. Appl. Environ. Microbiol. 58:1760-1763
8. King, E.B. Parke, J.L. 1993. Biocontrol of Aphanomyces root rot and Pythium damping-off by
Pseudomonas cepacia AMMD on four pea cultivars. Plant Dis. 77:1185-1188.
9. Cartwright, D.K. Benson, D.M. 1995. Comparison of
Pseudomonas species and application techniques for biocontrol of Rhizoctonia stem rot of poinsettia. Plant Dis. 79: 309-313.
10. Mao, W. Lewis, J.A. Hebbar, P.K. Lumsden, R.D. 1997. Seed treatment with a fungal or a bacterial antagonist for reducing corn damping-off caused by species of
Pythium and
Fusarium. [Erratum: July 1997, v. 81 (7), p. 824.] Plant Dis. 81: 450-454
11. Milus, E. A. and Rothrock, C. S. 1997. Efficacy of bacterial seed treatments for controlling Pythium root rot of winter wheat. Plant Dis. 81:180-184.
12. Joy, A. E. and Parke, J. L. 1994. Biocontrol of Alternaria leaf blight on American ginseng by
Burkholderia cepacia AMMD. Pages 93-100 in: Challenges of the 21st century. Proceedings of the International Ginseng Conference, Vancouver, B.C. (Bailey, W. G., Whitehead, C., Proctor, J. T. A., Kyle, J. T., eds.) Simon Fraser Univ., Burnaby, B.C. Canada.
13. Janisiewicz, W. Yourman, L. Roitman, J. Mahoney, N. 1991. Postharvest control of blue mold and gray mold of apples and pears by dip treatment with pyrrolnitrin, a metabolite of
Pseudomonas cepacia. Plant Dis.:490-494.
14. Smilanick, J.L. Denis-Arrue, R. 1992. Control of green mold of lemons with
Pseudomonas species. Plant Dis. 76:481-485.
15. Smilanick, J.L. Denis-Arrue, R. Bosch, J.R. Gonzalez, A.R., Henson, D. Janisiewicz, W.J. 1993. Control of postharvest brown rot of nectarines and peaches by
Pseudomonas species. Crop Prot. 12:513-520.
16. Roitman, J.N. Mahoney, N.E. Janisiewicz, W.J. 1990. Production and composition of phenylpyrrole metabolites produced by
Pseudomonas cepacia. Appl. Microbiol. Biotech. 34:381-386.
17. Rosales, A. M., Thomashow, L., Cook, R. J., and Mew, T. W. 1995. Isolation and identification of antifungal metabolites produced by rice-associated antagonistic
Pseudomonas spp. Phytopathology 85:1028-1032.
18. Govan, J., Hughes, J., Vandamme, P. 1996.
Burkholderia cepacia: medical, taxonomic, and ecological issues. J. Med. Microbiol. 45:395-407.
19. Govan, J., Brown, P., Maddison, J., Doherty, C., Nelson, J., Dodd, M. et al. 1993. Evidence for transmission of
Pseudomonas cepacia by social contact in cystic fibrosis. Lancet 342:15-19.
20. Gonzalez, C.F. Pettit, E.A. Valadez, V.A. Provin, E.M. 1997. Mobilization, cloning, and sequence determination of a plasmid-encoded polygalacturonase from a phytopathogenic
Burkholderia (Pseudomonas) cepacia. MPMI 10:840-85.
21. Fisher, M. C., LiPuma, J. J., Dasen, S. E., Caputo, G. C., Mortensen, J. E., McGowan, K. L., and Stull, T. L. 1993. Source of
Pseudomonas cepacia: ribotyping of isolates from patients and from the environment. J. Pediatr. 123:745-747.
22. Yohalem, D. S. and Lorbeer, J. W. 1994. Intraspecific metabolic diversity among strains of
Burkholderia cepacia isolated from decayed onions, soils, and the clinical environment. Antonie van Leewenhoek 65:111-131.
23. Govan, J. R. and Harris, G. 1985. Typing of
Pseudomonas cepacia by bacteriocin susceptibility and production. J. Clin. Microbiol. 4:490-494.
24. Butler, S. L., Doherty, C. J., Hughes, J. E., Nelson, J. W., and Govan, J. R. 1995.
Burkholderia cepacia and cystic fibrosis: do natural environments present a potential hazard? J. Clin. Microbiol. 4:1001-1004.
25. Mahenthiralingam, E., Campbell, M. E., Henry, D. A., and Speert, D. P. 1996. Epidemiology of
Burkholderia cepacia infection in patients with cystic fibrosis: analysis by random amplified polymorphic DNA (RAPD) fingerprinting. J. Clin. Microbiol. 34:2914-2920.
26. Vandamme, P., Holmes, B., Vancanneyt, M., Coenye, T., Hoste, B., Coopman, R., et al. 1997. Occurrence of multiple genomovars of
Burkholderia cepacia in cystic fibrosis patients and proposal of
Burkholderia multivorans sp. nov. Int. J. Syst. Bacteriol. 47:1188-1200.
27. Mahenthiralingam, E., Bischof, J., Byrne, S. K., Radomski, C., Davies, J. E., Av-Gay, Y., Vandamme, P. 2000.DNA-based diagnostic approaches for identification of
Burkholderia cepacia complex,
Burkholderia vietnamiensis, Burkholderia multivorans,
Burkholderia stabilis and
Burkholderia cepacia genomovars I and III.
J Clin. Microbiol. 38 :3615-3173.
28. Coenye, T. Mahenthiralingam, E. and Vandamme, P. 2000. Taxonomy of
B. cepacia-like biocontrol strains. International
Burkholderia cepacia Working Group, April 7-9, Bethesda, Maryland. Abstract.
29. Coenye,T., LiPuma, J.J., Henry, D., Hoste, B., Vandemeulebroecke, K., Gillis, M., Speert, D. P. & Vandamme, P. 2000.
Burkholderia cepacia genomovar VI, a new member of the
Burkholderia cepacia complex isolated from cystic fibrosis patients.
Int. J. Syst.
Evol. Microbiol. (in press)
30. Sajjan, U. S., Sun, L., Goldstein, R., and Forstner, J. F. 1995. Cable (Cbl) type II pili of cystic fibrosis-associated
Burkholderia (Pseudomonas) cepacia: nucleotide sequence of the
cblA major subunit pilin gene and novel morphology of the assembled appendage fibers. J. Bacteriol. 177:1030-1038.
31. Mahenthiralingam, E., Simpson, D. A., and Speert, D. P. 1997. Identification and characterization of a novel DNA marker associated with epidemic
Burkholderia cepacia strains recovered from patients with cystic fibrosis. J. Clin. Microbiol. 35:808-816.
32. Coenye, T. & Vandamme, P. 2003. Diversity and significance of
Burkholderia species occupying diverse ecological niches.
Env Microbiol 5:719-729.
33. Payne, G.W., Vandamme, P., Morgan, S.H., LiPuma, J.J., Coenye, T., Weightman, A.J., Jones, H. & Mahenthiralingam, E. 2005. Expansion of a
recA gene based identification approach to include the entire
Burkholderia genus.
Appl Env Microbiol 71:3917-3927.
34. Vermis, K., Coenye, T., LiPuma, J.J., Mahenthiralingam, E., Nelis, H.J. & Vandamme, P. 2004. Proposal to accommodate
Burkholderia cepacia genomovar VI as
Burkholderia dolosa sp. nov.
Int J Syst Evol Microbiol 54:689-691.
35. Vandamme, P., Holmes, B., Coenye, T., Goris, J., Mahenthiralingam, E., LiPuma, J.J. & Govan, J.R.W. 2003.
Burkholderia cenocepacia sp. nov. - a new twist to an old story.
Res Microbiol 154:91-96.