L. C. Galvez, K. Korus, J. Fernandez, and J. L. Behn,
Department of Plant Pathology, and
N. Banjara, Department of Agronomy,
University of Nebraska Lincoln, Lincoln, NE 68583-0722
Galvez, L.C., Korus, K., Fernandez, J., Behn, J.L., and Banjara, N. 2010. The Threat of Pierce’s Disease to
Midwest Wine and Table Grapes. Online. APSnet Features. doi:10.1094/APSnetFeature-2010-1015.
Grape production in the United States is expanding rapidly, especially as new growers are starting vineyards in many regions of the country. Commercial wine grape production in the Midwest, along with production in California, Oregon, and New York, has increased along with the demand for high-quality wine. There are more than 652 commercial wineries throughout the Midwest region (11,17), defined by the U.S. Census Bureau as the 12 states in the central and northeastern United States. The number of wineries in the United States has steadily increased over the past twenty years and currently exceeds 6000 (36).
The wine industry has expanded considerably in recent years in the Midwest (15,17). This increase can be attributed to the growing demands for wine both in local and international markets. Ohio has the most wineries of the Midwest states (Table 1). Wineries are considered a new industry in Minnesota, where there were 3 wineries in 1997, expanding to 36 wineries at present (21). Nebraska and surrounding states are developing rapidly as table grape and wine producers. Wisconsin had 24 wineries in the early 2000 and now has around 50 wineries (66). Kansas, South Dakota, and North Dakota have the least number of wineries, with 17, 14, and 5, respectively (17). This increase in wineries corresponds to the increase in vineyards to meet the demands for wine production.
Table 1. Winery numbers in the Midwest United States (14,16).
State |
1990-2000 |
2005 |
2009 |
Ohio |
43 |
92 |
118 |
Michigan |
25 |
93 |
100 |
Missouri |
30 |
66 |
95 |
Illinois |
12 |
58 |
80 |
Iowa |
13 |
36 |
71 |
Wisconsin |
NA |
24 |
49 |
Indiana |
27 |
30 |
42 |
Minnesota |
3 |
17 |
36 |
Nebraska |
4 |
13 |
25 |
Kansas |
NA |
10 |
17 |
South Dakota |
NA |
10 |
14 |
North Dakota |
NA |
4 |
5 |
Total |
− |
453 |
652 |
NA = data not available.
Grape cultivars grown in the Midwest are selected on the basis of vine hardiness, fruit characteristics, ripening time, tolerance to diseases and insects, vine characteristics, market outlet, and requirements of the processor or consumer who will purchase the crop. The comparison of popular grape cultivars grown in the Midwest to those of California reveals almost no overlap (Table 2). Some of these varieties show differences in relative susceptibility to powdery mildew (Erysiphe necator), Botrytis bunch rot and blight (Botrytis cinerea), Phomopsis cane and leaf spot (Phomopsis viticola), Eutypa dieback (Eutypa lata), and crown gall (Agrobacterium vitis). Only a few of the cultivars grown in the Midwest have been tested for resistance to Xylella fastidiosa, the causal agent of Pierce’s Disease [(49) as cited by (63)].
Table 2. Comparison of grape cultivars grown in California and the Midwest US.
Location |
Table Grapes |
Wine Grapes |
Midwestx |
DeChaunac Concord Esprit Edelweiss Frontenac(MN 1047) Jupiter LaCrescent (MN 1166) Leon Millot Mars Reliance St. Croix Swenson Red Vidal blanc Vignoles |
Baco noir Cabernet Franc Catbernet Sauvignon Catawba Cayuga white Chambourcin Chancellor Chardonel Chardonnay DeChaunac Delaware Edelwiess Esprit Frontenac |
LaCrosse Leon Millot Limberger Marechal Foch Melody Niagara Seyval blanc St. Pepin Traminette Ventura Vidal Blanc Vignoles Reisling St. Croix |
Californiay |
Thompson Seedless Flame Seedless Ruby Seedless Perlette Autumn Royal Crimson Seedless Red Globe Fantasy Seedless Beauty Seedless Marroo Seedless Sugraone Calmeria Princess
|
Arneis Barbera Cabernet Franc Cabernet Sauvignon Charbono Chardonnay Chenin Blanc Cinsault Dolcetto French Colombard Gamay Gewurztraminer Grenache Noir Lenoir Malbec Marsanne Merlot |
Mourvedre Muscat Nebbiolo Petite Sirah Pinot Blanc Pinot Gris Pinot Grigio Riesling Roussanne Sauvignon Blanc Scheurebe Sémillon Syrah Viognier Sangiovese Zinfandel |
x Midwest Grape Production Guide, 2005.
y California Winery Advisor, 2009.
Xylella fastidiosa (Xf) is a fastidious gram-negative, xylem-limited bacterium associated with a large number of diseases including Pierce’s Disease, phony peach disease, plum leaf scald, citrus variegated chlorosis, scorch of almond, oleander leaf scorch, and several other diseases (2,10,29,38,54). This bacterium has a very wide host range and has been reported to cause diseases in over 100 plant species of both monocots and dicots (29). Different subspecies or strains of Xf can cause disease in different plants, and in some cases the bacterium can inhabit the plant without causing symptoms. The fastidious bacterium resides in the plant xylem tissue or water conducting vessels and is spread exclusively by xylem-feeding insects known as sharpshooter leafhoppers (26). Pierce’s Disease is known to be prevalent within the United States from Florida to California, and in other countries throughout Central and South America.
In 1892, Newton Pierce was the first plant pathologist to describe the "mysterious vine disease" eventually known as Pierce’s disease (PD). Since the 1880s, Xf has been reported to cause considerable losses in Southern California, destroying more than 35,000 acres of vineyards. As a result, nearly all viticulture was forced to move northward due to the inability to produce a quality grape in this area due to PD.
The term "fastidiosa" indicates Xf is difficult to recover from host plant xylem, primarily because it does not grow on common bacterial media (64). However, there has been some recent success in isolation and culturing of certain Xf strains. Although Xf is classified as a single species, there is a complex and poorly defined relationship between hosts and strains of this unique pathogen (27). Currently there are several Xf strains that, based on the 16S-23S intergenic spacer region, were grouped under several subspecies (50). Xf subsp. multiplex causes disease in peach, plum, almond, elm, pigeon grape, sycamore, and other trees. Xf subsp. pauca causes disease in citrus and coffee, while Xf subsp. fastidiosa causes disease in grape, alfalfa, almond, and maple (50). Using the same basis for classification, Hernandez-Martinez et al. (23) reclassified Xf into four subspecies with the addition of Xf subsp. sandyi, which causes disease in oleander, daylily, jacaranda, and magnolia. They also proposed that strains isolated from mulberry with mulberry leaf scorch (MLS), which belong to Xf subsp. fastidiosa (9), be grouped into a separate subspecies because of their genetic differences from Xf subsp. fastidiosa and because MLS strains did not infect grapes or oleander. On the other hand, a classification scheme using fluorescent amplified-fragment length polymorphism (fAFLP) revealed a strain that infects mulberry, grapes, and temecula, and belongs to the Xf subsp fastidiosa group (31). Thus, different methods of classification revealed grape-infecting strains in different Xf subspecies groups.
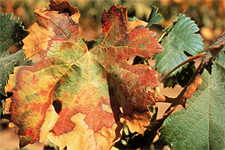 Fig. 1. Symptoms of Pierce's disease in grapevines caused by Xylella fastidiosa (courtesy A.C. Goheen; reproduced from Compendium of Grape Diseases, 1988, American Phytopathological Society, St. Paul, MN). |
|
Typical PD symptoms begin to appear in grapevines during late summer when weather conditions are predominately hot and dry or when grape plants are exposed to drought stress. Plants infected with Xf exhibit distinct symptoms consisting of marginal leaf necrosis, leaf blade drop, leaf scorch, shriveled berries, dieback, and death (29) (Fig. 1). Infected leaves display marginal chlorosis as well as chlorosis adjacent to dead tissue. The drying and scorching of leaves progresses for a few days or even weeks until they eventually drop, leaving their petioles attached to the vine and creating a "matchstick" appearance. Bacterial cells attach to the xylem vessel and multiply, forming biofilm-like colonies that occlude the xylem, disrupting water transport throughout different parts of the plant (6). This blockage in water flow is exacerbated by gum deposits, tyloses or deposition of other bacterial- or plant-derived compounds (37,59). Symptom development begins about 3 months or as long as 18 months after initial infection. Pierce’s disease bacterial strains currently are limited to the warmer climates in the United States. Climatic differences between regions can affect the timing and severity of symptoms, but not the type of symptoms. Warm climates accelerate symptom development because moisture stress in the plant can be severe even with adequate soil moisture.
The Vectors. For quite some time, PD was thought to be caused by an insect-borne virus because of its transmissibility via xylem-feeding insect vectors and via grafting (24). Similarly, no fungi or bacteria were isolated from PD infected plants (25). The reemergence of PD in the Southern California vineyards enabled researchers to identify xylem-feeding vectors such as sharpshooter leafhoppers belonging to the subfamily Cicadellinae (51) and spittlebugs from the family Cercopidae (14).
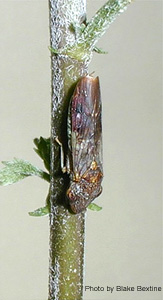 Fig. 2. The glassy-winged sharpshooter leafhopper vector of Xylella fasidiosa (courtesy B. Bextine, Fletcher and Wayadande, 2002, The Plant Health Instructor). |
|
In coastal California, the principal vector of PD was Graphocephala atropunctata, known as the blue-green sharpshooter (BGSS) (52). BGSS overwinters in riparian vegetation but readily feeds and multiplies on grapevines. These insects transmit Xf in a persistent manner, with a short or even no latent period required between acquisition and transmission (44). In the southeastern USA, another sharpshooter, Homalodisca vitripennis Germar (Fig. 2) (61), was identified as a vector of Xf. Homalodisca vitripennis [formerly Homalodisca coagulata (60)], popularly known as the glassy-winged sharpshooter (GWSS), was introduced to California in the 1990s. BGSS and GWSS have similar feeding habits. However, BGSS prefers to feed on young leaves and tissues while GWSS, although it can exist on these tissues, is also found on woody parts of the plants (29). At present, GWSS is considered to be the most efficient vector of PD because it displays greater mobility, is capable of feeding on older woody stems, and has a wider host range than the native sharpshooters (33). Its establishment in California threatens the overall wine grape, table grape, and almond industries (48).
Pathogen-Vector-Host Interaction. Three events are essential for the successful transmission of the pathogen to the plant. First is the acquisition of the pathogen by a vector from a diseased host plant. Acquisition efficiency is directly proportional to the population of the bacteria from the infected hosts (26,48). Similarly, efficiency of acquisition also depends upon the sharpshooter feeding site whether it be leaves, stems, or older woody branches, however, no report suggests a preferred feeding site by the vector (26). The second event is the attachment or retention of the acquired bacteria into the vector foregut. De la Fuente and co-workers (13) suggested that Type I and Type IV pili are involved in the attachment to the cuticle within an environment described as a "fast fluid flow" condition (13). Microscopy studies revealed that the bacteria form a biofilm in the foregut of the sharpshooters where they are polarly attached to the cuticle, with the cuticle being shed at each molt (43). Lastly, the pathogen must be detached and introduced into a new host during vector feeding. The lack of a latent period suggests that the population of bacteria within the vector is not a significant factor affecting the transmission efficiency (1) (Fig. 3).
Chatterjee and co-workers (7) used green flourescent protein (GFP)-tagging of Xf to monitor the correlation between the colonized vessels and symptom expression. They found a five-fold increase in colonized vessels from symptomatic leaves compared to symptomless leaves (7,42). It was also observed that vessels in symptomatic leaves contained large bacterial colonies while asymptomatic leaves from infected plants had vessels that contained very small colonies of bacterial cells. This finding suggested that vessel blockage determined symptom expression.
Like many plant pathogenic bacteria, Xf harbors a cell-to-cell signaling system which plays an important role in colonization, pathogenesis, and biofilm formation (62). A set of genes known for regulation of pathogenic factor (rpf) is required for the synthesis of diffusible signaling factor (DSF), involved in the formation of biofilm in the foregut of the vector (7). Rpf was also involved in colonization of both the vector and the host (43) and pathogenesis in the host (7). Genomic characterization of Xf also revealed its richness in adhesins and hemaglutinin-encoding genes that are involved in the interaction with vectors and hosts, and cell-to-cell attachment in biofilm formation (8). Whether these virulence factors are secreted via Type I or Type II secretion systems is not yet clear, but sequence analysis reveals that Xf possesses genes for both Type I and Type II secretion systems for the export of exoenzymes involved in plant cell wall degradation and allow bacterial colonization of plant xylem (30,53).
Disease Detection. Preliminary PD diagnosis is commonly done by symptom identification. However, visual diagnosis is not definitive due to the similarities of PD symptoms to those of other grape diseases, with the possible exception of the "matchstick" symptom indicator of Xf infected grapevines (32). Leaf scorching, the typical PD symptom, is absent during the early stages of infection (28), and there are inconsistencies in the correlation between symptom expression and bacterial populations in the leaf (18). Thus, limitations of visual diagnosis can lead to incorrect disease management decisions and may increase the likelihood of disease spread and dispersal. Reliable diagnosis is dependent upon the use of diagnostic techniques such as bacterial culturing, enzyme-linked immunosorbent assay (ELISA), and polymerase chain reaction (PCR). However, each method has its own limitations. Culturing the pathogen is a difficult task especially during the early season, presumably due to sampling and low population numbers. ELISA is very useful for late season identification of PD but the test is not reliable for early season diagnosis (56), presumably for the same reason of low population numbers. ELISA, however, is still commonly used because of its capability for large scale detection at a lower cost. PCR is an efficient technique for Xf detection due to the availability of PCR primers that target different specific components of the genome, particularly the 16S rRNA region (8). Different PCR-based techniques are being evaluated to determine the most economic, sensitive, fast, and reliable method of early PD detection. Currently, the most sensitive, cost effective method is the use of real-time PCR with the use of sap flow during bud break. This technique gets rid of the intricacy of DNA extraction and the inhibitory compounds that limit sensitive detection (N. Schaad, personal communication). Clearly, the reliability of the various diagnostic techniques depends on the sample, timing, and plant tissue tested.
The primary factor to consider with respect to the probability of PD development in the Midwest is climate. In the years since the discovery of PD, reports of its presence have been restricted to areas with a sub-tropical climate. In the United States, its distribution has been mostly limited to the southernmost grape growing states and west coast, with the greatest distribution and greatest disease severity occurring in Florida and California. The correlation between disease incidence and the concentration of vineyards may be coincidental. However, this pattern of disease seclusion to sub-tropical climates appears to be a result of the intolerance of Xf and its respective vectors to cold temperatures. Even though Xf is favored by a sub-tropical climate, it also is sensitive to elevated temperatures, and growth above 34ºC does not occur (16).
There are no reports of any of the known insect vectors of Xf being present in the Midwest, including Homalodisca vitripennis (syn. coagulata), Draeculacephala minerva, Xyphon fulgida, Graphocephala coccinea, G. versuta, and G. atropunctata (IFAS, Global Invasive Species Database). However, the Midwest is home to many related xylem feeding, hemipteran insects. Many of the known vectors of Xf increase their numbers and overwinter on lush grasslands, riparian buffer strips, and alfalfa fields, which also are prevalent in the Midwest. Of further concern is the observation that alfalfa may be an alternate host for Xf (55).
Many xylem feeding insects found throughout the Midwest are known to feed on the younger, greener tissues of their respective host plants. Theoretically, if Xf and a suitable vector were introduced into the Midwest, the insect would likely also feed on the younger tissues. If Xf is introduced to these parts of the grape vine, disease severity is expected to be low and overwintering inside the plant hindered because of vine pruning practices. The risk of disease in the Midwest, aside from the effect of temperature, relies on two important parameters: the introduction of Xf; and the association between Xf and a xylem-feeding insect pest of grapes.
Little work has been done to correlate the effects of severe cold weather, such as seen in the Midwest during a typical winter, and its effect on the survival of either H. vitripennis or Xf. However, there is a relationship between low temperatures (~5ºC) and the corresponding growth rate of Xf. Henneberger and Stevenson (22) isolated Xf from the roots and shoots of sycamore trees (a natural alternate host) at various times throughout the year at varying temperatures. They constructed a plot correlating the frequency of Xf isolation with the respective temperature when the isolation was attempted which revealed a decrease in isolation success as the temperature approached 0ºC. These data suggest that survival of Xf is minimal at temperatures below freezing. In a similar study, Feil and Purcell (16) found a similar correlation between temperature and the growth rate of Xf. They were able to show that, in vitro, the optimal growth conditions for this bacterium ranged from 25 to 32ºC and that growth declined and was even suppressed when temperatures fell below 12ºC.
The above findings conflict with the discovery of a Xf strain that causes mulberry leaf scorch (9). Based on restriction fragment length polymorphism (RFLP) and random amplified polymorphic DNA (RAPD) molecular genome assays, it was found that this strain is closely related to the subspecies that causes PD. Furthermore, the mulberry trees from which the bacterium was isolated were growing naturally in two different locations, namely Massachusetts and Nebraska, suggesting that this particular strain has adapted to the harsh temperature fluctuations of the Midwest and Northeast.
More recently, a report from Oklahoma revealed that the table grape cultivar ‘Concord’ displayed typical symptoms of PD. Real-time PCR analysis of DNA isolated from vine samples using available specific primers coupled with ELISA confirmed the presence of Xf (57). This finding in Oklahoma has caused concern among Midwest wineries because of its geographical proximity. The recent identification of Xf in grapes suggests the possibility that the strain of Xf infecting woody trees was able to overcome previous grape cultivar resistance. Currently there are no studies to investigate the ability of this strain to infect any of the widely grown grape cultivars throughout the Midwest. Until this is done, it will not be possible to extrapolate the possibility of PD on the Midwestern grape and wine industry.
Climate Change Adaptations. Over the last 100 years average temperatures across the northern Midwest have increased ca. 4ºF (2ºC), and average temperatures have decreased in the southern Midwest by 1ºF (0.5ºC). These trends are projected to continue (39). A shift to warmer temperatures throughout the Midwest may enable establishment of organisms that would otherwise be unable to exist in these areas. An increase in the minimum temperature by 10ºF (12ºC) might shift a particular region’s climate such that freezing would be avoided altogether. The pathogen and vector may also be able to adapt in the presence or absence of climate change. Rising temperatures would also lengthen the growing season, and potentially increase drought conditions throughout the Midwest. In combination, these elements could enable an increased proliferation of insect pests and pathogens. Drought stress not only increases the probability of PD incidence, but may also increase the expression of disease symptoms on stressed plants due to compromised defense system. In fact, McElrone et al. (34) revealed that occlusion of the xylem vessels by Xf was exaggerated when host plants experienced drought stress (19,34).
There are several disease management strategies used with some success in areas with severe PD. These include host resistance, vector control, and changes in cultural practices. Used in combination as an integrated pest management strategy, they should prove to be fairly successful in preventing the infection and spread of Xf in Midwestern vineyards.
The most popular grape cultivars of European and American descent are susceptible to PD to some degree. However, there are a few native species found in the southeastern part of the USA that have shown resistance to Xf. Muscadine grape (Vitis rotundifolia) cultivars have varying degrees of resistance to X. fastidiosa, but are not immune to the pathogen. Although some muscadine grape cultivars may be highly resistant to PD, they lack the fruit quality that most popular cultivars offer. Also, Vitis vinifera cultivars have shown differences in PD resistance in California, with ‘Petit Sirah,’ ‘Chenin blanc,’ and ‘Sylvaner’ among the most resistant cultivars (29). These cultivars may be useful for resistance gene introduction into popular wine and table grape cultivars.
Potential transgenic grapes resistant to PD are highly controversial and are not currently considered within the grape industry (40). Wine-making, an "Old World" industry based on historical European wine regions, carries with it original wine grape names representing different cultivars, tradition, and prestige. The general opposition to genetically altering the classic cultivars is that they will not be the same cultivar anymore, hence losing their status among the top wine-making grapes. Plant breeders and the skeptical wine consumers are at a standstill, involving regulatory and marketing issues, with the growers left in the middle, scrambling for a better solution to PD. If resistance genes can be identified and introduced into a desirable but susceptible grape genome, new cultivars may be produced having varied levels of resistance to PD which may or may not be accepted in the trade.
With management of any mobile pest, such as the GWSS, timing is crucial. Targeting the first generation of GWSS is most effective in controlling Xf transmission (29). These studies have shown that an early insecticide application before the appearance of the first generation of GWSS helps to slow PD spread. Insecticides may also need to be applied to surrounding vegetation containing hosts of GWSS and other insect vectors of Xf. Although effective for shorter annual growth periods, it is less effective in areas with longer growing seasons and warmer climates, such as those experienced in Florida (29). Because systemic insecticides last a few months inside plants, a second application may be needed in areas where the growing season is extended.
Several insecticides (i.e., foliar-applied acetamiprid and soil-applied imidacloprid and thiamethoxam) have been tested for control of GWSS and other Xf transmitting insects (3). The most effective insecticides for insect control, inhibiting bacterial transmission, and PD spread are the neonicotinoids (1). These insecticides are systemic and aid in disrupting the H. vitripennis-X. fastidiosa interface.
Pierce’s Disease of grapes has recently been reported in Oklahoma, adjacent to the lower Midwest region. While PD is unlikely to become a serious problem in the near future, this could change with adaptation of both the vector and the pathogen to colder climates and introduction of susceptible cultivars. With a warmer climate predicted for the Midwest, it is also possible that climate would not be a limiting factor in the future. This review of the current status reveals that growers, consultants and investigators associated with grape and wine production are at some risk of PD and should plan accordingly. Such plans should include periodic surveys, determination of susceptibility of current cultivars and potential forward breeding for resistance to this potentially devastating disease.
This review is one of the final requirements of the course Plant Path 867. The authors were all enrolled in this course, and wish to acknowledge their professors Drs. Anne Vidaver and Gary Yuen and Drs. Fred Baxendale, Robert Harveson, Paul Read, for their valuable comments and suggestions on the manuscript.
1. Almeida, R. P. P., Blua, M. J., Lopes, J. R. S., and Purcell, A. H. 2005. Vector transmission of Xylella fastidiosa: Applying fundamental knowledge to generate disease management strategies. Annu. Ent. Soc. Amer. 98:775-786.
2. Almeida, R. P. P., Nascimento, F. E., Chau, J., Prado, S. S., Tsai, C., Lopes, S. A., and Lopes, J. R. S. 2008. Genetic structure and biology of Xylella fastidiosa causing disease in citrus and coffee in Brazil. Appl. Environ. Microbiol. 74:3690-3701. doi:10.1128/AEM.02388-07.
3. Bethke, J. A., Blua, M. J., and Redak, R. A. 2001. Effect of selected insecticides on Homalodisca coagulata (Homoptera: Cicadellidae) and transmission of oleander leaf scorch in a greenhouse study. J. Econo. Entomol. 94:1031-1036.
4. California Winery Advisor. 2009. Wine grape varieties. Online. Winery Advisor LLC, San Luis Obispo, CA.
5. Carbajal, D., and Morano, L. 2004. Variation of Xylella fastidiosa colonization in tolerant and susceptible grape cultivars. Abstract.
6. Chatterjee, S., Almeida, R. P. P., and Lindow, S. E. 2008. Living in two worlds: The plant and insect lifestyles of Xylella fastidiosa. Annu. Rev. Phytopathol. 46:243-271.
7. Chatterjee, S., Newman, K. L., and Lindow, S. E. 2008. Cell-to-cell signaling in Xylella fastidiosa suppresses movement and xylem vessel colonization in grape. Mol. Plant-Microbe Interact. 21:1309-1315.
8. Chen, J., Groves, E. L., Civerolo, M., Viveres, M., Freeman, M., and Zheng, Y. 2005. Two Xylella fastidiosa genotypes associated with almond leaf scorch disease on the same location in California. Phytopathol. 95:708-714.
9. Chen, J., Hartung, J. S., Chang, C., and Vidaver, A. K. 2002. An evolutionary perspective of Pierce's disease of grapevine, citrus variegated chlorosis, and mulberry leaf scorch diseases. Curr. Microbiol. 45:423-428. DOI: 10.1007/s00284-002-3785-7
10. Costa, H. S. 2004. Incidence of Xylella fastidiosa in Landscape Plants. Dec. 15, 2004, Turf and Landscape Institute, Ontario, CA.
11. Dami, I., Bordelon, B., Ferree, D. C., Brown, M., Ellis, M. A., Williams, R. N., Doohan, D. 2005. Midwest Grape Production Guide. Ext. Bulletin 919-05, Ohio State Univ., Columbus, OH.
12. Davis, M. J., Purcell, A. H. and Thomson, S. V. 1978. Pierce’s disease of grapevines: Isolation of the causal bacterium. Science 199:75-77.
13. De La Fuente, L., Burr, T. J., and Hoch, H. C. 2007. Mutations in type I and type IV pilus biosynthetic genes affect twitching motility rates in Xylella fastidiosa. J. Bacteriol. 189:7507-7510.
14. DeLong, D. M., and Severin, H. H. P. 1950. Spittle-insect vectors of Pierce’s disease virus I. Characters, distribution, and food plants. Hilgardia 19:339-356.
15. Eckstein, C. 2009. Ohio Grape Industry Committee Presentation. Northwest Agribusiness Breakfast Forum. July 16, 2009.
16. Feil, H., and Purcell, A. H. 2001. Temperature-dependent growth and survival of Xylella fastidiosa in vitro and in potted grapevines. Plant Dis. 85:1230-1234.
17. Fisher, C. 2010. Number of U.S. wineries reaches 6,223. Online. Wine Business Monthly, Sonoma, CA.
18. Gambetta, G. A., Fei, J., Rost, T. L., and Matthews, M. A. 2007. Leaf scorch symptoms are not correlated with bacterial populations during Pierce’s disease. J. Exp. Bot. 58:4037-4046.
19. Garrett, K. A., Dendy, S. P., Frank, E. E., Rouse, M. N., and Travers, S. E. 2006. Climate change effects on plant disease: Genomes to ecosystems. Annu. Rev. Phytopathol. 44:489-509.
20. Gartner, W., and Tuck, B. 2008. The economic contribution of grape growers and wineries to the state of Minnesota. Dept. of Applied Economics, Univ. of Minnesota, St. Paul, MN.
21. Hagen, D. 2010. Recession hasn't slowed Minnesota's vineyards. The Land Online, Mankato, MN.
22. Henneberger, T. S. M., Stevenson, K. L., Britton, K. O., and Chang, C. J. 2004. Distribution of Xylella fastidiosa in sycamore associated with low temperature and host resistance. Plant Dis. 88:951-958.
23. Hernendez-Martinez, R., de la Cerda, K. A., Costa, H. S., Cooksey, D. A., and Wong, F. P. 2007. Phylogenetic relationships of Xylella fastidiosa strains isolated from landscape ornamentals in southern California. Phytopathol. 97:857-864.
24. Hewitt, W. B. 1939. A transmissible disease of grapevines. Phytopathol. 29:10.
25. Hewitt, W. B., Frazier, N. W, and Freitag, J. H. 1949. Pierce’s disease investigations. Hilgardia 19:207-264.
26. Hill, B. L., and Purcell, A. H. 1997. Populations of Xylella fastidiosa in plants required for transmission by an efficient vector. Phytopathol. 87:1197-1201.
27. Hopkins, D. L. 1989. Xylella fastidiosa: Xylem-limited bacterial pathogen of plants. Ann. Rev. Phytopathol. 27:271-290.
28. Hopkins, D. L. 1981. Seasonal concentration of the Pierce’s disease bacterium in grapevine stems, petioles, and leaf veins. Phytopathol. 71:415-418.
29. Hopkins, D. L., and Purcell, A. H. 2002. Xylella fastidiosa: Cause of Pierce’s disease of grapevine and other emergent diseases. Plant Dis. 86:1056-1066.
30. Jha, G., Rajeshwari, R., and Sonti, R. V. 2005. Bacterial type two secretion system secreted proteins: double-edged swords for plant pathogens. Mol. Plant-Microbe Interact. 18:891-898.
31. Kishi, L. T., Wickert, E., and Lemos, E. G. D. 2008. Evaluation Of Xylella fastidiosa Genetic Diversity by Faflp Markers. Rev. Bras. Frutic., Jaboticabal - SP. 30:202-208.
32. Krell, R. K., Perring, T. M., Farrar, C. A., Park, Y. L., and Gispert, C. 2006. Intraplant sampling of grapevines for Pierce’s disease diagnosis. Plant Dis. 90:351-357.
33. Larsen, D. 2000. Glassy-winged sharpshooter update - 9/00. Online. GWSS Features, American Vineyard Magazine, Malcolm Media, Clovis CA.
34. McElrone, A. J., Sherald, J. L., and Forseth, I. N. 2003. Interactive effects of water stress and xylem-limited bacterial infection on the water relations of a host vine. J. Exp. Bot. 54:419-430.
35. Michigan Grape and Wine Industry Council. 2002. Fast Facts. MGWIC, Lansing, MI.
36. Midwest Grape and Wine Industry Institute. 2008. Leopold Center Advisory Board Meeting. March 4, 2008. Des Moines, IA.
37. Mollenhauer, H. H., and Hopkins, D. L. 1976. Xylem morphology of Pierce’s disease-infected grapevines with different levels of tolerance. Physiol. Plant Pathol. 9:95-100.
38. Montero-Astua, M., Saborio, R, G., Chacon-Diaz, C., Villalobos, W., Moreira, L., Rivera, C., and Hartung, J. S. 2008. First report of Xylella fastidiosa in avocado in Costa Rica. Plant Dis. 92:175.
39. National Assessment Synthesis Team: 2000. Climate Change Impacts in the U.S.: Overview. Cambridge Univ. Press, Cambridge, UK.
40. National Research Council. 2004. California Agricultural Research Priorities: Pierce’s Disease. National Academics Press, Washington, DC.
41. Nebraska Winery and Grapes Growers Association. 2007. Strategic plan. NWGGA, Lincoln, NE.
42. Newman, K. L., Almeida, R. P. P., Purcell, A. H., and Lindow, S. E. 2003. Use of a green fluorescent strain for analysis of Xylella fastidiosa colonization of Vitis vinifera. Appl. Environ. Microbiol. 69:7319-7327.
43. Newman, K. L., Almeida, R. P. P., Purcell, A. H., and Lindow, S. E. 2004. Cell-cell signaling controls Xylella fastidiosa interactions with both insects and plants. Proc. Natl. Acad. Sci. U.S.A. 101:1737-1742.
44. Purcell, A. H., and Finlay, A. H. 1979. Evidence for noncirculative transmission of Pierce's disease bacterium by sharpshooter leafhoppers. Phytopathol. 69:393-395.
45. Purcell, A. H., and Hopkins, D. L. 1996. Fastidious xylem-limited bacterial plant pathogens. Annu. Rev. Phytopathol. 34:131-151.
46. Quade, K. 2010. The great grapes of Nebraska. L: Lincoln’s Premier Lifestyle Magazine 8:22-25.
47. Read, P. 2004. Developing a grape and wine industry in a non-traditional region. ISHS Acta Hort. 652:493-496.
48. Redak, R. A., Purcell, A. H., Lopes, J. R. S., Blua, M. J., Mizell III, R. F., and. Anderson, P. C. 2004. The biology of xylem fluid-feeding insect vectors of Xylella fastidiosa and their relation to disease epidemiology. Annu. Rev. Entomol. 49:243-270.
49. Reisch, B. I., Pool, R. M., Peterson, D. V., Martens, M. H., and Henick-Kling, T. 1993. Wine and juice grape varieties for cool climates. Coop. Ext. Info. Bull. 233, Cornell Univ., Ithaca, NY.
50. Schaad, N. W., Postnikova, E., Lacy, G., M'Barek, F., and Chang, C. J. 2004. Xylella fastidiosa subspecies: X. fastidiosa subsp. piercei, subsp. nov., X. fastidiosa subsp. multiplex subsp. nov., and X. fastidiosa subsp. pauca subsp. nov. Syst. Appl. Microbiol. 27:290-300.
51. Severin, H. H. P. 1950. Spittle-insect vectors of Pierce’s disease virus II. Life history and virus transmission. Hilgardia 19:357-81.
52. Severin, H. H. P. 1949. Transmission of the virus of Pierce’s disease of grapevines by leafhoppers. Hilgardia 19:190-206.
53. Simpson, A. J. G., Reinach, F. C., Arruda, P., Abreu, F. A., Acencio, M., Alvarenga, R., Alves, L. M. C., Araya, J. E., Baia, G. S., Baptista, C. S., et al. 2000. The genome sequence of the plant pathogen Xylella fastidiosa. Nature 406:151-159.
54. Singh, R., Ferrin, D. M., and Huang, Q. 2010. First report of Xylella fastidiosa associated with Oleander leaf scorch in Louisiana. Plant Dis. 94:274.
55. Sisterson, M. S., Thimmiraju, S. R., Daane, K., and Groves, R. L. 2008. Assessment of the role of alfalfa in the spread of Xylella fastidiosa in California. Phytopathol. 98:S147-S147.
56. Smart, C. D., Hendson, M., Guilhabert, M. R., Saunders, S., Friebertshauser, G., Purcell, A. H., and Kirkpatrick, B. C. 1998. Seasonal detection of Xylella fastidiosa in grapevines with culture, ELISA and PCR. Phytopathol. 88:S83.
57. Smith, D. L., Dominiak-Olson, J., and Sharber, C. D. 2009. First report of Pierce's disease of grape caused by Xylella fastidiosa in Oklahoma. Plant Dis. 93:762.
58. SDSU Agricultural Experiment Station. 2010. Viticulture in South Dakota. Online. Agr. Exp. Station, Coll. of Agr. and Biol. Sci., South Dakota State Univ., Brookings, SD.
59. Stevenson, J. F., Labavitch, J. M., Matthews, M. A., and Rost, T. L. 2004. Grapevine susceptibility to Pierce’s disease II: Progression of anatomical symptoms. Am. J. Enol. Vitic. 55:238-245.
60. Takiya, D. M., McKamey, S. H., and Cavichioli, R. R. 2006. Validity of Homalodisca and of H. vitripennis as the name for glassy-winged sharpshooter (Hemiptera: Cicadellidae: Cicadellinae). Annu. Entomol. Soc. Amer. 99:648-655.
61. Turner, W. F., and Pollard, H. N. 1959. Life histories and behavior of five insect vectors of phony peach disease. Tech. Bull. 1188, USDA, Washington, DC.
62. von Bodman, S. B., Bauer, W. D., and Coplin, D. L. 2003. Quorum sensing in plant-pathogenic bacteria. Annu. Rev. Phytopathol. 41:455-482.
63. Walker, A., Tenscher, A., Riaz, S., and Ramming, D. 2009. Breeding Pierce’s disease resistant winegrapes. Online. Pages 8-12 in: FPS Grape Program Newsletter, October 2009. Foundation Plant Services, Univ. of California, Davis, CA.
64. Wells, J. M., Raju, B. C., Hung, H. Y., Weisburg, W. G., Mandelco-Paul, L., and Brenner, D. J. 1987. Xylella fastidiosa new genus new species gram-negative xylem-limited fastidious plant bacteria related to Xanthomonas spp. Int. J. Syst. Bacteriol. 37:136-143.
65. Wine Making in the Midwest, Fast Fact. 2008. Chicago and Midwest Wine Show. Sept. 27-29, 2008.
66. Wine Trail Traveler. 2010. Winery and vineyard virtual visitor center: Wisconsin. Online. Wine Trail Traveler LLC, Columbia, MD.